Improved use of a public good selects for the evolution of undifferentiated multicellularity
- PMID: 23577233
- PMCID: PMC3614033
- DOI: 10.7554/eLife.00367
Improved use of a public good selects for the evolution of undifferentiated multicellularity
Abstract
We do not know how or why multicellularity evolved. We used the budding yeast, Saccharomyces cerevisiae, to ask whether nutrients that must be digested extracellularly select for the evolution of undifferentiated multicellularity. Because yeast use invertase to hydrolyze sucrose extracellularly and import the resulting monosaccharides, single cells cannot grow at low cell and sucrose concentrations. Three engineered strategies overcame this problem: forming multicellular clumps, importing sucrose before hydrolysis, and increasing invertase expression. We evolved populations in low sucrose to ask which strategy they would adopt. Of 12 successful clones, 11 formed multicellular clumps through incomplete cell separation, 10 increased invertase expression, none imported sucrose, and 11 increased hexose transporter expression, a strategy we had not engineered. Identifying causal mutations revealed genes and pathways, which frequently contributed to the evolved phenotype. Our study shows that combining rational design with experimental evolution can help evaluate hypotheses about evolutionary strategies. DOI:http://dx.doi.org/10.7554/eLife.00367.001.
Keywords: Evolution of cooperation; Experimental evolution; Multicellularity; S. cerevisiae.
Conflict of interest statement
The authors declare that no competing interests exist.
Figures


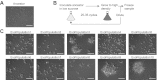
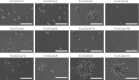
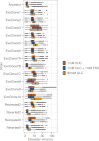
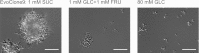
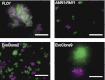
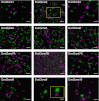
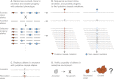
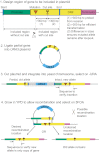
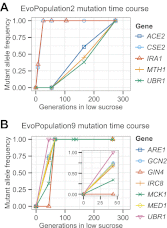
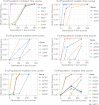
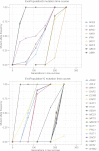
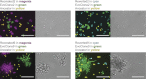
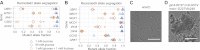
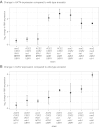
Comment in
-
A sticky solution.Elife. 2013 Apr 2;2:e00655. doi: 10.7554/eLife.00655. Elife. 2013. PMID: 23577236 Free PMC article.
Similar articles
-
A sticky solution.Elife. 2013 Apr 2;2:e00655. doi: 10.7554/eLife.00655. Elife. 2013. PMID: 23577236 Free PMC article.
-
Sucrose utilization in budding yeast as a model for the origin of undifferentiated multicellularity.PLoS Biol. 2011 Aug;9(8):e1001122. doi: 10.1371/journal.pbio.1001122. Epub 2011 Aug 9. PLoS Biol. 2011. PMID: 21857801 Free PMC article.
-
Engineering topology and kinetics of sucrose metabolism in Saccharomyces cerevisiae for improved ethanol yield.Metab Eng. 2011 Nov;13(6):694-703. doi: 10.1016/j.ymben.2011.09.005. Epub 2011 Sep 22. Metab Eng. 2011. PMID: 21963484
-
[Invertase Overproduction May Provide for Inulin Fermentation by Selection Strains of Saccharomyces cerevisiae].Mikrobiologiia. 2015 Mar-Apr;84(2):160-4. Mikrobiologiia. 2015. PMID: 26263621 Review. Russian.
-
Carbon source dependent promoters in yeasts.Microb Cell Fact. 2014 Jan 9;13:5. doi: 10.1186/1475-2859-13-5. Microb Cell Fact. 2014. PMID: 24401081 Free PMC article. Review.
Cited by
-
Clonal yeast biofilms can reap competitive advantages through cell differentiation without being obligatorily multicellular.Proc Biol Sci. 2016 Nov 16;283(1842):20161303. doi: 10.1098/rspb.2016.1303. Proc Biol Sci. 2016. PMID: 27807261 Free PMC article.
-
Regulation of sedimentation rate shapes the evolution of multicellularity in a close unicellular relative of animals.PLoS Biol. 2022 Mar 29;20(3):e3001551. doi: 10.1371/journal.pbio.3001551. eCollection 2022 Mar. PLoS Biol. 2022. PMID: 35349578 Free PMC article.
-
A Zpr1 co-chaperone mediates folding of eukaryotic translation elongation factor 1A via a GTPase cycle.Mol Cell. 2023 Sep 7;83(17):3108-3122.e13. doi: 10.1016/j.molcel.2023.07.028. Epub 2023 Aug 18. Mol Cell. 2023. PMID: 37597513 Free PMC article.
-
The spectrum of adaptive mutations in experimental evolution.Genomics. 2014 Dec;104(6 Pt A):412-6. doi: 10.1016/j.ygeno.2014.09.011. Epub 2014 Sep 28. Genomics. 2014. PMID: 25269377 Free PMC article. Review.
-
Optogenetic Tools for Control of Public Goods in Saccharomyces cerevisiae.mSphere. 2021 Aug 25;6(4):e0058121. doi: 10.1128/mSphere.00581-21. Epub 2021 Aug 25. mSphere. 2021. PMID: 34431694 Free PMC article.
References
-
- Agresti A, Coull B. 1998. Approximate is better than ‘exact' for interval estimation of binomial proportions. Am Stat 52:119–26
Publication types
MeSH terms
Substances
Grants and funding
LinkOut - more resources
Full Text Sources
Other Literature Sources
Molecular Biology Databases