A mammalian pre-mRNA 5' end capping quality control mechanism and an unexpected link of capping to pre-mRNA processing
- PMID: 23523372
- PMCID: PMC3630477
- DOI: 10.1016/j.molcel.2013.02.017
A mammalian pre-mRNA 5' end capping quality control mechanism and an unexpected link of capping to pre-mRNA processing
Abstract
Recently, we reported that two homologous yeast proteins, Rai1 and Dxo1, function in a quality control mechanism to clear cells of incompletely 5' end-capped messenger RNAs (mRNAs). Here, we report that their mammalian homolog, Dom3Z (referred to as DXO), possesses pyrophosphohydrolase, decapping, and 5'-to-3' exoribonuclease activities. Surprisingly, we found that DXO preferentially degrades defectively capped pre-mRNAs in cells. Additional studies show that incompletely capped pre-mRNAs are inefficiently spliced at all introns, a fact that contrasts with current understanding, and are also poorly cleaved for polyadenylation. Crystal structures of DXO in complex with substrate mimic and products at a resolution of up to 1.5Å provide elegant insights into the catalytic mechanism and molecular basis for their three apparently distinct activities. Our data reveal a pre-mRNA 5' end capping quality control mechanism in mammalian cells, indicating DXO as the central player for this mechanism, and demonstrate an unexpected intimate link between proper 5' end capping and subsequent pre-mRNA processing.
Copyright © 2013 Elsevier Inc. All rights reserved.
Figures
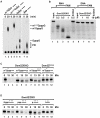
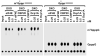
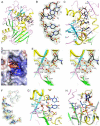
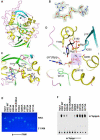
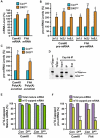
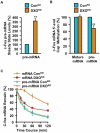
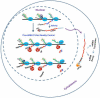
Comment in
-
Judge, jury, and executioner: DXO functions as a decapping enzyme and exoribonuclease in pre-mRNA quality control.Mol Cell. 2013 Apr 11;50(1):2-4. doi: 10.1016/j.molcel.2013.03.025. Mol Cell. 2013. PMID: 23582258 Free PMC article.
Similar articles
-
Dxo1 is a new type of eukaryotic enzyme with both decapping and 5'-3' exoribonuclease activity.Nat Struct Mol Biol. 2012 Oct;19(10):1011-7. doi: 10.1038/nsmb.2381. Epub 2012 Sep 9. Nat Struct Mol Biol. 2012. PMID: 22961381 Free PMC article.
-
A novel 5'-hydroxyl dinucleotide hydrolase activity for the DXO/Rai1 family of enzymes.Nucleic Acids Res. 2020 Jan 10;48(1):349-358. doi: 10.1093/nar/gkz1107. Nucleic Acids Res. 2020. PMID: 31777937 Free PMC article.
-
DXO/Rai1 enzymes remove 5'-end FAD and dephospho-CoA caps on RNAs.Nucleic Acids Res. 2020 Jun 19;48(11):6136-6148. doi: 10.1093/nar/gkaa297. Nucleic Acids Res. 2020. PMID: 32374864 Free PMC article.
-
mRNA quality control at the 5' end.J Zhejiang Univ Sci B. 2014 May;15(5):438-43. doi: 10.1631/jzus.B1400070. J Zhejiang Univ Sci B. 2014. PMID: 24793761 Free PMC article. Review.
-
Recent insights into noncanonical 5' capping and decapping of RNA.J Biol Chem. 2022 Aug;298(8):102171. doi: 10.1016/j.jbc.2022.102171. Epub 2022 Jun 21. J Biol Chem. 2022. PMID: 35750211 Free PMC article. Review.
Cited by
-
Hypo- and Hyper-Assembly Diseases of RNA-Protein Complexes.Trends Mol Med. 2016 Jul;22(7):615-628. doi: 10.1016/j.molmed.2016.05.005. Epub 2016 Jun 3. Trends Mol Med. 2016. PMID: 27263464 Free PMC article. Review.
-
RNA Cap Methyltransferase Activity Assay.Bio Protoc. 2018 Mar 20;8(6):e2767. doi: 10.21769/BioProtoc.2767. Bio Protoc. 2018. PMID: 29644259 Free PMC article.
-
Myc and mRNA capping.Biochim Biophys Acta. 2015 May;1849(5):501-5. doi: 10.1016/j.bbagrm.2014.03.007. Epub 2014 Mar 27. Biochim Biophys Acta. 2015. PMID: 24681440 Free PMC article. Review.
-
Upregulation of NPL4 promotes bladder cancer cell proliferation by inhibiting DXO destabilization of cyclin D1 mRNA.Cancer Cell Int. 2019 May 30;19:149. doi: 10.1186/s12935-019-0874-2. eCollection 2019. Cancer Cell Int. 2019. PMID: 31164795 Free PMC article.
-
RNA guanine-7 methyltransferase catalyzes the methylation of cytoplasmically recapped RNAs.Nucleic Acids Res. 2017 Oct 13;45(18):10726-10739. doi: 10.1093/nar/gkx801. Nucleic Acids Res. 2017. PMID: 28981715 Free PMC article.
References
-
- Brunger AT, Adams PD, Clore GM, DeLano WL, Gros P, Grosse-Kunstleve RW, Jiang JS, Kuszewski J, Nilges M, Pannu NS, et al. Crystallography & NMR system: A new software suite for macromolecular structure determination. Acta Crystallogr D Biol Crystallogr. 1998;54:905–921. - PubMed
-
- Calero G, Wilson KF, Ly T, Rios-Steiner JL, Clardy JC, Cerione RA. Structural basis of m7GpppG binding to the nuclear cap-binding protein complex. Nat Struct Biol. 2002;9:912–917. - PubMed
-
- Colot HV, Stutz F, Rosbash M. The yeast splicing factor Mud13p is a commitment complex component and corresponds to CBP20, the small subunit of the nuclear cap-binding complex. Genes Dev. 1996;10:1699–1708. - PubMed
Publication types
MeSH terms
Substances
Associated data
- Actions
- Actions
- Actions
Grants and funding
LinkOut - more resources
Full Text Sources
Other Literature Sources
Molecular Biology Databases
Miscellaneous