Postnatal loss of P/Q-type channels confined to rhombic-lip-derived neurons alters synaptic transmission at the parallel fiber to purkinje cell synapse and replicates genomic Cacna1a mutation phenotype of ataxia and seizures in mice
- PMID: 23516282
- PMCID: PMC3641643
- DOI: 10.1523/JNEUROSCI.5442-12.2013
Postnatal loss of P/Q-type channels confined to rhombic-lip-derived neurons alters synaptic transmission at the parallel fiber to purkinje cell synapse and replicates genomic Cacna1a mutation phenotype of ataxia and seizures in mice
Abstract
Ataxia, episodic dyskinesia, and thalamocortical seizures are associated with an inherited loss of P/Q-type voltage-gated Ca(2+) channel function. P/Q-type channels are widely expressed throughout the neuraxis, obscuring identification of the critical networks underlying these complex neurological disorders. We showed recently that the conditional postnatal loss of P/Q-type channels in cerebellar Purkinje cells (PCs) in mice (purky) leads to these aberrant phenotypes, suggesting that intrinsic alteration in PC output is a sufficient pathogenic factor for disease initiation. The question arises whether P/Q-type channel deletion confined to a single upstream cerebellar synapse might induce the pathophysiological abnormality of genomically inherited P/Q-type channel disorders. PCs integrate two excitatory inputs, climbing fibers from inferior olive and parallel fibers (PFs) from granule cells (GCs) that receive mossy fiber (MF) input derived from precerebellar nuclei. In this study, we introduce a new mouse model with a selective knock-out of P/Q-type channels in rhombic-lip-derived neurons including the PF and MF pathways (quirky). We found that in quirky mice, PF-PC synaptic transmission is reduced during low-frequency stimulation. Using focal light stimulation of GCs that express optogenetic light-sensitive channels, channelrhodopsin-2, we found that modulation of PC firing via GC input is reduced in quirky mice. Phenotypic analysis revealed that quirky mice display ataxia, dyskinesia, and absence epilepsy. These results suggest that developmental alteration of patterned input confined to only one of the main afferent cerebellar excitatory synaptic pathways has a significant role in generating the neurological phenotype associated with the global genomic loss of P/Q-type channel function.
Figures
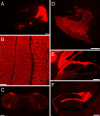
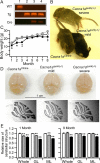
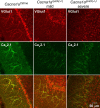
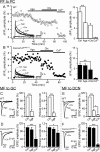
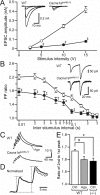
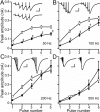
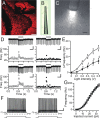
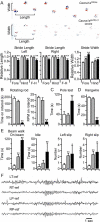
Similar articles
-
Delayed postnatal loss of P/Q-type calcium channels recapitulates the absence epilepsy, dyskinesia, and ataxia phenotypes of genomic Cacna1a mutations.J Neurosci. 2011 Mar 16;31(11):4311-26. doi: 10.1523/JNEUROSCI.5342-10.2011. J Neurosci. 2011. PMID: 21411672 Free PMC article.
-
Impact of the leaner P/Q-type Ca2+ channel mutation on excitatory synaptic transmission in cerebellar Purkinje cells.J Physiol. 2008 Sep 15;586(18):4501-15. doi: 10.1113/jphysiol.2008.156232. Epub 2008 Jul 31. J Physiol. 2008. PMID: 18669535 Free PMC article.
-
Bidirectional alterations in cerebellar synaptic transmission of tottering and rolling Ca2+ channel mutant mice.J Neurosci. 2002 Jun 1;22(11):4388-98. doi: 10.1523/JNEUROSCI.22-11-04388.2002. J Neurosci. 2002. PMID: 12040045 Free PMC article.
-
Activity-dependent maturation of climbing fiber to Purkinje cell synapses during postnatal cerebellar development.Cerebellum. 2012 Jun;11(2):449-50. doi: 10.1007/s12311-011-0337-3. Cerebellum. 2012. PMID: 22194041 Review.
-
[P/Q-type voltage-dependent calcium channels in neurological disease].Neurologia. 2007 Oct;22(8):511-6. Neurologia. 2007. PMID: 17573560 Review. Spanish.
Cited by
-
Current Opinions and Areas of Consensus on the Role of the Cerebellum in Dystonia.Cerebellum. 2017 Apr;16(2):577-594. doi: 10.1007/s12311-016-0825-6. Cerebellum. 2017. PMID: 27734238 Free PMC article.
-
Spinocerebellar ataxia type 6 protein aggregates cause deficits in motor learning and cerebellar plasticity.J Neurosci. 2015 Jun 10;35(23):8882-95. doi: 10.1523/JNEUROSCI.0891-15.2015. J Neurosci. 2015. PMID: 26063920 Free PMC article.
-
Molecular Mechanisms Underlying the Generation of Absence Seizures: Identification of Potential Targets for Therapeutic Intervention.Int J Mol Sci. 2024 Sep 11;25(18):9821. doi: 10.3390/ijms25189821. Int J Mol Sci. 2024. PMID: 39337309 Free PMC article. Review.
-
Abnormal synaptic Ca(2+) homeostasis and morphology in cortical neurons of familial hemiplegic migraine type 1 mutant mice.Ann Neurol. 2015 Aug;78(2):193-210. doi: 10.1002/ana.24449. Epub 2015 Jul 6. Ann Neurol. 2015. PMID: 26032020 Free PMC article.
-
Reversing frontal disinhibition rescues behavioural deficits in models of CACNA1A-associated neurodevelopment disorders.Mol Psychiatry. 2021 Dec;26(12):7225-7246. doi: 10.1038/s41380-021-01175-1. Epub 2021 Jun 14. Mol Psychiatry. 2021. PMID: 34127816
References
-
- Bosman LW, Konnerth A. Activity-dependent plasticity of developing climbing fiber-Purkinje cell synapses. Neuroscience. 2009;162:612–623. - PubMed
-
- Brozoski TJ, Ciobanu L, Bauer CA. Central neural activity in rats with tinnitus evaluated with manganese-enhanced magnetic resonance imaging (MEMRI) Hear Res. 2007;228:168–179. - PubMed
Publication types
MeSH terms
Substances
Grants and funding
LinkOut - more resources
Full Text Sources
Other Literature Sources
Molecular Biology Databases
Research Materials
Miscellaneous