Negative regulation of 26S proteasome stability via calpain-mediated cleavage of Rpn10 subunit upon mitochondrial dysfunction in neurons
- PMID: 23508964
- PMCID: PMC3636900
- DOI: 10.1074/jbc.M113.464552
Negative regulation of 26S proteasome stability via calpain-mediated cleavage of Rpn10 subunit upon mitochondrial dysfunction in neurons
Abstract
Proteasomal and mitochondrial dysfunctions are implicated in chronic neurodegenerative diseases. To investigate the impact of mitochondrial impairment on the proteasome, we treated rat cerebral cortical neurons with oligomycin, antimycin, or rotenone, which inhibit different elements of the electron transport chain. Firstly, we observed a reduction in ubiquitinated proteins and E1 activity. Secondly, we established that 26S proteasomes are disassembled with a decline in activity. Thirdly, we show, to our knowledge for the first time, that calpain activation triggers the selective processing of the 26S proteasome subunit Rpn10. Other proteasome subunits tested were not affected. Calpain also cleaved caspase 3 to an inactive fragment, thus preventing apoptosis that is an energy-dependent cell death pathway. In addition, calpain cleaved the microtubule-associated protein Tau, a major component of neurofibrillary tangles in Alzheimer disease and other tauopathies. Fourthly, we detected a rise in 20S proteasome levels and activity. Finally, we show that both acute (16 h) and long term (up to 7 days) mitochondrial impairment led to down-regulation of ubiquitinated-proteins, 26S proteasome disassembly, and a rise in 20S proteasomes. We postulate that upon mitochondrial dysfunction, ATP depletion and calpain activation contribute to the demise of protein turnover by the ubiquitin/proteasome pathway. The concomitant rise in 20S proteasomes, which seem to degrade proteins in an unregulated and energy-independent manner, in the short term may carry out the turnover of randomly unfolded oxidized proteins. However, if chronic, it could lead to neurodegeneration as regulated protein degradation by the ubiquitin/proteasome pathway is essential for neuronal survival.
Figures
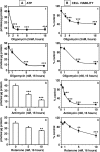
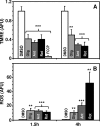
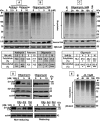
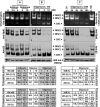
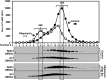
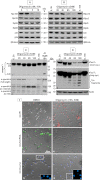
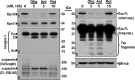
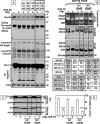
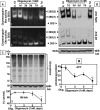
Similar articles
-
Neurotoxic mechanisms by which the USP14 inhibitor IU1 depletes ubiquitinated proteins and Tau in rat cerebral cortical neurons: Relevance to Alzheimer's disease.Biochim Biophys Acta Mol Basis Dis. 2017 Jun;1863(6):1157-1170. doi: 10.1016/j.bbadis.2017.03.017. Epub 2017 Apr 1. Biochim Biophys Acta Mol Basis Dis. 2017. PMID: 28372990 Free PMC article.
-
Coordination between proteasome impairment and caspase activation leading to TAU pathology: neuroprotection by cAMP.Cell Death Dis. 2012 Jun 21;3(6):e326. doi: 10.1038/cddis.2012.70. Cell Death Dis. 2012. PMID: 22717581 Free PMC article.
-
Mitochondrial and calcium perturbations in rat CNS neurons induce calpain-cleavage of Parkin: Phosphatase inhibition stabilizes pSer65Parkin reducing its calpain-cleavage.Biochim Biophys Acta Mol Basis Dis. 2019 Jun 1;1865(6):1436-1450. doi: 10.1016/j.bbadis.2019.02.016. Epub 2019 Feb 21. Biochim Biophys Acta Mol Basis Dis. 2019. PMID: 30796971 Free PMC article.
-
Substrate receptors of proteasomes.Biol Rev Camb Philos Soc. 2018 Nov;93(4):1765-1777. doi: 10.1111/brv.12419. Epub 2018 May 6. Biol Rev Camb Philos Soc. 2018. PMID: 29732666 Review.
-
The complexity of recognition of ubiquitinated substrates by the 26S proteasome.Biochim Biophys Acta. 2014 Jan;1843(1):86-96. doi: 10.1016/j.bbamcr.2013.07.007. Epub 2013 Jul 18. Biochim Biophys Acta. 2014. PMID: 23872423 Review.
Cited by
-
Renal cold storage followed by transplantation impairs proteasome function and mitochondrial protein homeostasis.Am J Physiol Renal Physiol. 2019 Jan 1;316(1):F42-F53. doi: 10.1152/ajprenal.00316.2018. Epub 2018 Oct 10. Am J Physiol Renal Physiol. 2019. PMID: 30303714 Free PMC article.
-
Selenite activates the alternative oxidase pathway and alters primary metabolism in Brassica napus roots: evidence of a mitochondrial stress response.BMC Plant Biol. 2014 Sep 30;14:259. doi: 10.1186/s12870-014-0259-6. BMC Plant Biol. 2014. PMID: 25267309 Free PMC article.
-
DNA-controlled protein fluorescence: Design of aptamer-split peptide hetero-modulator for GFP to respond to intracellular ATP levels.Nucleic Acids Res. 2024 Aug 12;52(14):8063-8071. doi: 10.1093/nar/gkae532. Nucleic Acids Res. 2024. PMID: 38917331 Free PMC article.
-
Efficiency of the four proteasome subtypes to degrade ubiquitinated or oxidized proteins.Sci Rep. 2020 Sep 25;10(1):15765. doi: 10.1038/s41598-020-71550-5. Sci Rep. 2020. PMID: 32978409 Free PMC article.
-
Molecular events underlying Parkinson's disease - an interwoven tapestry.Front Neurol. 2013 Apr 8;4:33. doi: 10.3389/fneur.2013.00033. eCollection 2013. Front Neurol. 2013. PMID: 23580245 Free PMC article.
References
-
- Livnat-Levanon N., Glickman M. H. (2011) Ubiquitin-proteasome system and mitochondria – reciprocity. Biochim. Biophys. Acta 1809, 80–87 - PubMed
-
- Ding Q., Dimayuga E., Keller J. N. (2006) Proteasome regulation of oxidative stress in aging and age-related diseases of the CNS. Antioxid. Redox Signal. 8, 163–172 - PubMed
-
- Reddy P. H. (2007) Mitochondrial dysfunction in aging and Alzheimer's disease: strategies to protect neurons. Antioxid. Redox Signal. 9, 1647–1658 - PubMed
-
- Beal M. F. (2005) Mitochondria take center stage in aging and neurodegeneration. Ann. Neurol. 58, 495–505 - PubMed
Publication types
MeSH terms
Substances
Grants and funding
LinkOut - more resources
Full Text Sources
Other Literature Sources
Molecular Biology Databases
Research Materials