Sclerotial formation of Polyporus umbellatus by low temperature treatment under artificial conditions
- PMID: 23437090
- PMCID: PMC3577777
- DOI: 10.1371/journal.pone.0056190
Sclerotial formation of Polyporus umbellatus by low temperature treatment under artificial conditions
Abstract
Background: Polyporus umbellatus sclerotia have been used as a diuretic agent in China for over two thousand years. A shortage of the natural P. umbellatus has prompted researchers to induce sclerotial formation in the laboratory.
Methodology/principal finding: P. umbellatus cultivation in a sawdust-based substrate was investigated to evaluate the effect of low temperature conditions on sclerotial formation. A phenol-sulfuric acid method was employed to determine the polysaccharide content of wild P. umbellatus sclerotia and mycelia and sclerotia grown in low-temperature treatments. In addition, reactive oxygen species (ROS) content, expressed as the fluorescence intensity of mycelia during sclerotial differentiation was determined. Analysis of ROS generation and sclerotial formation in mycelia after treatment with the antioxidants such as diphenyleneiodonium chloride (DPI), apocynin (Apo), or vitamin C were studied. Furthermore, macroscopic and microscopic characteristics of sclerotial differentiation were observed. Sclerotia were not induced by continuous cultivation at 25°C. The polysaccharide content of the artificial sclerotia is 78% of that of wild sclerotia. In the low-temperature treatment group, the fluorescent intensity of ROS was higher than that of the room temperature (25°C) group which did not induce sclerotial formation all through the cultivation. The antioxidants DPI and Apo reduced ROS levels and did not induce sclerotial formation. Although the concentration-dependent effects of vitamin C (5-15 mg mL(-1)) also reduced ROS generation and inhibited sclerotial formation, using a low concentration of vitamin C (1 mg mL(-1)) successfully induced sclerotial differentiation and increased ROS production.
Conclusions/significance: Exposure to low temperatures induced P. umbellatus sclerotial morphogenesis during cultivation. Low temperature treatment enhanced ROS in mycelia, which may be important in triggering sclerotial differentiation in P. umbellatus. Moreover, the application of antioxidants impaired ROS generation and inhibited sclerotial formation. Our findings may help to provide new insights into the biological mechanisms underlying sclerotial morphogenesis in P. umbellatus.
Conflict of interest statement
Figures
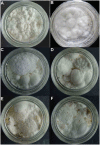
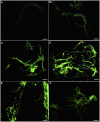
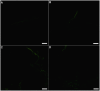
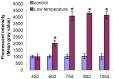
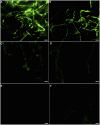
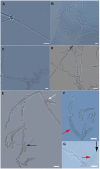
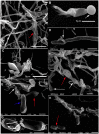
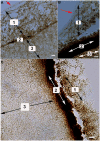
Similar articles
-
Nox gene expression and cytochemical localization of hydrogen peroxide in Polyporus umbellatus sclerotial formation.Int J Mol Sci. 2013 Nov 20;14(11):22967-81. doi: 10.3390/ijms141122967. Int J Mol Sci. 2013. PMID: 24264041 Free PMC article.
-
Oxalic acid and sclerotial differentiation of Polyporus umbellatus.Sci Rep. 2015 Jun 1;5:10759. doi: 10.1038/srep10759. Sci Rep. 2015. PMID: 26030006 Free PMC article.
-
Nutritional factors determining sclerotial formation of Polyporus umbellatus.Lett Appl Microbiol. 2009 Aug;49(2):283-8. doi: 10.1111/j.1472-765X.2009.02656.x. Epub 2009 Jun 10. Lett Appl Microbiol. 2009. PMID: 19515145
-
[Correlative analysis advance of chemical constituents of Polyporus umbellatus and Armillaria mellea].Zhongguo Zhong Yao Za Zhi. 2017 Aug;42(15):2905-2914. doi: 10.19540/j.cnki.cjcmm.20170512.009. Zhongguo Zhong Yao Za Zhi. 2017. PMID: 29139256 Review. Chinese.
-
Polysaccharides from Polyporus umbellatus: A review on their extraction, modification, structure, and bioactivities.Int J Biol Macromol. 2021 Oct 31;189:124-134. doi: 10.1016/j.ijbiomac.2021.08.101. Epub 2021 Aug 19. Int J Biol Macromol. 2021. PMID: 34419536 Review.
Cited by
-
New Inonotus Polysaccharides: Characterization and Anticomplementary Activity of Inonotus rheades Mycelium Polymers.Polymers (Basel). 2023 Mar 1;15(5):1257. doi: 10.3390/polym15051257. Polymers (Basel). 2023. PMID: 36904498 Free PMC article.
-
Hypovirulence of Sclerotium rolfsii Caused by Associated RNA Mycovirus.Front Microbiol. 2016 Nov 10;7:1798. doi: 10.3389/fmicb.2016.01798. eCollection 2016. Front Microbiol. 2016. PMID: 27891121 Free PMC article.
-
PuCRZ1, an C2H2 transcription factor from Polyporus umbellatus, positively regulates mycelium response to osmotic stress.Front Microbiol. 2023 Apr 6;14:1131605. doi: 10.3389/fmicb.2023.1131605. eCollection 2023. Front Microbiol. 2023. PMID: 37089566 Free PMC article.
-
Transcriptome analysis of genes involved in defence response in Polyporus umbellatus with Armillaria mellea infection.Sci Rep. 2015 Nov 3;5:16075. doi: 10.1038/srep16075. Sci Rep. 2015. PMID: 26526032 Free PMC article.
-
Cloning and Expression Analysis of Bioluminescence Genes in Omphalotus guepiniiformis Reveal Stress-Dependent Regulation of Bioluminescence.Mycobiology. 2024 Jan 23;52(1):42-50. doi: 10.1080/12298093.2024.2302661. eCollection 2024. Mycobiology. 2024. PMID: 38415178 Free PMC article.
References
-
- Choi KD, Lee KT, Shim JO, Lee YS, Lee TS, et al. (2003) A new method for cultivation of sclerotium of Grifola umbellata . Mycobiology 31(2): 105–112.
-
- Guo WJ, Xing YM, Chen J, Guo SX (2011) Growth promoting effects of water extract of Armillaria mellea rhizomorph on mycelia of Polyporus umbellatus . Cryptogamie Mycol 32(2): 171–176.
-
- Xing YM, Chen J, Lv YL, Liang HQ, Guo SX (2011) Dertermination of optimal carbon source and pH value for sclerotial formation of Polyporus umbellatus under artificial conditions. Mycol Prog 10(1): 121–125.
-
- Zhao YY, Chao X, Zhang YM, Lin RC, Sun WJ (2010) Cytotoxic steroids from Polyporus umbellatus . Planta Med 76: 1755–1758. - PubMed
-
- Ohsawa T, Yukawa M, Takao C, Murayama M, Bando H (1992) Studies on constituents of fruit body of Polyporus umbellatus and their cytotoxic activity. Chem Pharm Bull 40: 143–147. - PubMed
Publication types
MeSH terms
Substances
Grants and funding
LinkOut - more resources
Full Text Sources
Other Literature Sources