Structural plasticity in the dentate gyrus- revisiting a classic injury model
- PMID: 23423628
- PMCID: PMC3575076
- DOI: 10.3389/fncir.2013.00017
Structural plasticity in the dentate gyrus- revisiting a classic injury model
Abstract
The adult brain is in a continuous state of remodeling. This is nowhere more true than in the dentate gyrus, where competing forces such as neurodegeneration and neurogenesis dynamically modify neuronal connectivity, and can occur simultaneously. This plasticity of the adult nervous system is particularly important in the context of traumatic brain injury or deafferentation. In this review, we summarize a classic injury model, lesioning of the perforant path, which removes the main extrahippocampal input to the dentate gyrus. Early studies revealed that in response to deafferentation, axons of remaining fiber systems and dendrites of mature granule cells undergo lamina-specific changes, providing one of the first examples of structural plasticity in the adult brain. Given the increasing role of adult-generated new neurons in the function of the dentate gyrus, we also compare the response of newborn and mature granule cells following lesioning of the perforant path. These studies provide insights not only to plasticity in the dentate gyrus, but also to the response of neural circuits to brain injury.
Keywords: adult neurogenesis; dentate gyrus; perforant path lesion; reactive synaptogenesis.
Figures
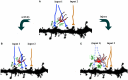
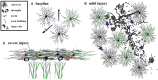
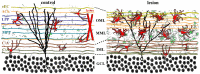
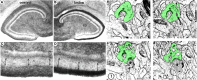
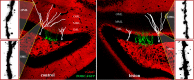
Similar articles
-
Neural injury alters proliferation and integration of adult-generated neurons in the dentate gyrus.J Neurosci. 2013 Mar 13;33(11):4754-67. doi: 10.1523/JNEUROSCI.4785-12.2013. J Neurosci. 2013. PMID: 23486947 Free PMC article.
-
Effects of adult-generated granule cells on coordinated network activity in the dentate gyrus.Hippocampus. 2012 Jan;22(1):106-16. doi: 10.1002/hipo.20860. Epub 2010 Sep 29. Hippocampus. 2012. PMID: 20882540 Free PMC article.
-
Excitability changes within transverse lamellae of dentate granule cells and their longitudinal spread following orthodromic or antidromic activation.Hippocampus. 2009 Jul;19(7):633-48. doi: 10.1002/hipo.20538. Hippocampus. 2009. PMID: 19115390
-
Adult neurogenesis and the plasticity of the dentate gyrus network.Eur J Neurosci. 2011 Mar;33(6):1055-61. doi: 10.1111/j.1460-9568.2011.07603.x. Eur J Neurosci. 2011. PMID: 21395848 Review.
-
Contributions of adult neurogenesis to dentate gyrus network activity and computations.Behav Brain Res. 2019 Nov 18;374:112112. doi: 10.1016/j.bbr.2019.112112. Epub 2019 Aug 1. Behav Brain Res. 2019. PMID: 31377252 Free PMC article. Review.
Cited by
-
Sensorimotor Intervention Recovers Noradrenaline Content in the Dentate Gyrus of Cortical Injured Rats.Neurochem Res. 2016 Dec;41(12):3261-3271. doi: 10.1007/s11064-016-2054-2. Epub 2016 Sep 17. Neurochem Res. 2016. PMID: 27639395
-
Structure, function, and plasticity of hippocampal dentate gyrus microcircuits.Front Neural Circuits. 2014 Sep 10;8:107. doi: 10.3389/fncir.2014.00107. eCollection 2014. Front Neural Circuits. 2014. PMID: 25309334 Free PMC article. No abstract available.
-
Layer-specific changes of KCC2 and NKCC1 in the mouse dentate gyrus after entorhinal denervation.Front Mol Neurosci. 2023 May 24;16:1118746. doi: 10.3389/fnmol.2023.1118746. eCollection 2023. Front Mol Neurosci. 2023. PMID: 37293543 Free PMC article.
-
Sphingosine-1-phosphate receptor inhibition prevents denervation-induced dendritic atrophy.Acta Neuropathol Commun. 2016 Mar 31;4:28. doi: 10.1186/s40478-016-0303-x. Acta Neuropathol Commun. 2016. PMID: 27036416 Free PMC article.
-
Heterogeneous CaMKII-Dependent Synaptic Compensations in CA1 Pyramidal Neurons From Acute Hippocampal Slices.Front Cell Neurosci. 2022 Mar 30;16:821088. doi: 10.3389/fncel.2022.821088. eCollection 2022. Front Cell Neurosci. 2022. PMID: 35431809 Free PMC article.
References
Publication types
MeSH terms
Grants and funding
LinkOut - more resources
Full Text Sources
Other Literature Sources