Characterization of fragile X mental retardation protein recruitment and dynamics in Drosophila stress granules
- PMID: 23408971
- PMCID: PMC3567066
- DOI: 10.1371/journal.pone.0055342
Characterization of fragile X mental retardation protein recruitment and dynamics in Drosophila stress granules
Abstract
The RNA-binding protein Fragile X Mental Retardation (FMRP) is an evolutionarily conserved protein that is particularly abundant in the brain due to its high expression in neurons. FMRP deficiency causes fragile X mental retardation syndrome. In neurons, FMRP controls the translation of target mRNAs in part by promoting dynamic transport in and out neuronal RNA granules. We and others have previously shown that upon stress, mammalian FMRP dissociates from translating polysomes to localize into neuronal-like granules termed stress granules (SG). This localization of FMRP in SG is conserved in Drosophila. Whether FMRP plays a key role in SG formation, how FMRP is recruited into SG, and whether its association with SG is dynamic are currently unknown. In contrast with mammalian FMRP, which has two paralog proteins, Drosophila FMR1 (dFMRP) is encoded by a single gene that has no paralog. Using this genetically simple model, we assessed the role of dFMRP in SG formation and defined the determinants required for its recruitment in SG as well as its dynamics in SG. We show that dFMRP is dispensable for SG formation in vitro and ex vivo. FRAP experiments showed that dFMRP shuttles in and out SG. The shuttling activity of dFMRP is mediated by a protein-protein interaction domain located at the N-terminus of the protein. This domain is, however, dispensable for the localization of dFMRP in SG. This localization of dFMRP in SG requires the KH and RGG motifs which are known to mediate RNA binding, as well as the C-terminal glutamine/asparagine rich domain. Our studies thus suggest that the mechanisms controlling the recruitment of FMRP into SG and those that promote its shuttling between granules and the cytosol are uncoupled. To our knowledge, this is the first demonstration of the regulated shuttling activity of a SG component between RNA granules and the cytosol.
Conflict of interest statement
Figures
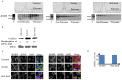
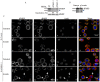
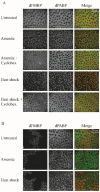
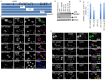
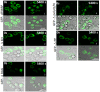
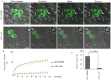
Similar articles
-
Characterization of Fragile X Mental Retardation Protein granules formation and dynamics in Drosophila.Biol Open. 2013 Jan 15;2(1):68-81. doi: 10.1242/bio.20123012. Epub 2012 Oct 31. Biol Open. 2013. PMID: 23336078 Free PMC article.
-
Proteomic analysis reveals CCT is a target of Fragile X mental retardation protein regulation in Drosophila.Dev Biol. 2010 Apr 15;340(2):408-18. doi: 10.1016/j.ydbio.2010.01.028. Epub 2010 Feb 1. Dev Biol. 2010. PMID: 20122915 Free PMC article.
-
Mutational analysis establishes a critical role for the N terminus of fragile X mental retardation protein FMRP.J Neurosci. 2008 Mar 19;28(12):3221-6. doi: 10.1523/JNEUROSCI.5528-07.2008. J Neurosci. 2008. PMID: 18354025 Free PMC article.
-
mRNPs, polysomes or granules: FMRP in neuronal protein synthesis.Curr Opin Neurobiol. 2006 Jun;16(3):265-9. doi: 10.1016/j.conb.2006.05.010. Epub 2006 May 16. Curr Opin Neurobiol. 2006. PMID: 16707258 Review.
-
Regulation of RNA granules by FMRP and implications for neurological diseases.Traffic. 2020 Jul;21(7):454-462. doi: 10.1111/tra.12733. Traffic. 2020. PMID: 32374065 Free PMC article. Review.
Cited by
-
FMRP cooperates with miRISC components to repress translation and regulate neurite morphogenesis in Drosophila.RNA Biol. 2024 Jan;21(1):11-22. doi: 10.1080/15476286.2024.2392304. Epub 2024 Aug 27. RNA Biol. 2024. PMID: 39190491 Free PMC article.
-
Mechanisms and Regulation of RNA Condensation in RNP Granule Formation.Trends Biochem Sci. 2020 Sep;45(9):764-778. doi: 10.1016/j.tibs.2020.05.002. Epub 2020 May 11. Trends Biochem Sci. 2020. PMID: 32475683 Free PMC article. Review.
-
Arginine methylation augments Sbp1 function in translation repression and decapping.FEBS J. 2019 Dec;286(23):4693-4708. doi: 10.1111/febs.15057. Epub 2019 Sep 23. FEBS J. 2019. PMID: 31495062 Free PMC article.
-
Gene and MicroRNA transcriptome analysis of Parkinson's related LRRK2 mouse models.PLoS One. 2014 Jan 10;9(1):e85510. doi: 10.1371/journal.pone.0085510. eCollection 2014. PLoS One. 2014. PMID: 24427314 Free PMC article.
-
FXS causing missense mutations disrupt FMRP granule formation, dynamics, and function.PLoS Genet. 2022 Feb 24;18(2):e1010084. doi: 10.1371/journal.pgen.1010084. eCollection 2022 Feb. PLoS Genet. 2022. PMID: 35202393 Free PMC article.
References
-
- Ashley CT, Sutcliffe JS, Kunst CB, Leiner HA, Eichler EE, et al. (1993) Human and murine FMR-1: alternative splicing and translational initiation downstream of the CGG-repeat. Nature genetics 4: 244–251. - PubMed
-
- Khandjian EW, Fortin A, Thibodeau A, Tremblay S, Cote F, et al. (1995) A heterogeneous set of FMR1 proteins is widely distributed in mouse tissues and is modulated in cell culture. Human molecular genetics 4: 783–789. - PubMed
-
- Siomi H, Siomi MC, Nussbaum RL, Dreyfuss G (1993) The protein product of the fragile X gene, FMR1, has characteristics of an RNA-binding protein. Cell 74: 291–298. - PubMed
-
- Santoro MR, Bray SM, Warren ST (2012) Molecular Mechanisms of Fragile X Syndrome: A Twenty-Year Perspective. Annual review of pathology 7: 219–245 doi:10.1146/annurev-pathol-011811–132457. - DOI - PubMed
Publication types
MeSH terms
Substances
Grants and funding
LinkOut - more resources
Full Text Sources
Other Literature Sources
Molecular Biology Databases
Miscellaneous