Depletion of PtdIns(4,5)P₂ underlies retinal degeneration in Drosophila trp mutants
- PMID: 23378018
- PMCID: PMC3635464
- DOI: 10.1242/jcs.120592
Depletion of PtdIns(4,5)P₂ underlies retinal degeneration in Drosophila trp mutants
Abstract
The prototypical transient receptor potential (TRP) channel is the major light-sensitive, and Ca(2+)-permeable channel in the microvillar photoreceptors of Drosophila. TRP channels are activated following hydrolysis of phosphatidylinositol 4,5-bisphosphate [PtdIns(4,5)P₂] by the key effector enzyme phospholipase C (PLC). Mutants lacking TRP channels undergo light-dependent retinal degeneration, as a consequence of the reduced Ca(2+) influx. It has been proposed that degeneration is caused by defects in the Ca(2+)-dependent visual pigment cycle, which result in accumulation of toxic phosphorylated metarhodopsin-arrestin complexes (MPP-Arr2). Here we show that two interventions, which prevent accumulation of MPP-Arr2, namely rearing under red light or eliminating the C-terminal rhodopsin phosphorylation sites, failed to rescue degeneration in trp mutants. Instead, degeneration in trp mutants reared under red light was rescued by mutation of PLC. Degeneration correlated closely with the light-induced depletion of PtdIns(4,5)P₂ that occurs in trp mutants due to failure of Ca(2+)-dependent inhibition of PLC. Severe retinal degeneration was also induced in the dark in otherwise wild-type flies by overexpression of a bacterial PtdInsPn phosphatase (SigD) to deplete PtdIns(4,5)P₂. In degenerating trp photoreceptors, phosphorylated Moesin, a PtdIns(4,5)P₂-regulated membrane-cytoskeleton linker essential for normal microvillar morphology, was found to delocalize from the rhabdomere and there was extensive microvillar actin depolymerisation. The results suggest that compromised light-induced Ca(2+) influx, due to loss of TRP channels, leads to PtdIns(4,5)P₂ depletion, resulting in dephosphorylation of Moesin, actin depolymerisation and disintegration of photoreceptor structure.
Figures
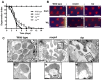
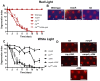
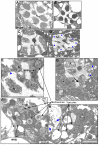
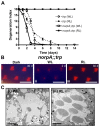
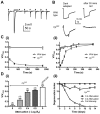
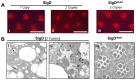
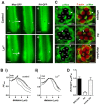
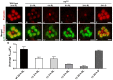
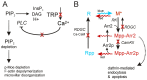
Similar articles
-
Photosensitive TRPs.Handb Exp Pharmacol. 2014;223:795-826. doi: 10.1007/978-3-319-05161-1_4. Handb Exp Pharmacol. 2014. PMID: 24961970 Review.
-
Exploring Excitotoxicity and Regulation of a Constitutively Active TRP Ca2+ Channel in Drosophila.Fly (Austin). 2021 Dec;15(1):8-27. doi: 10.1080/19336934.2020.1851586. Epub 2020 Dec 1. Fly (Austin). 2021. PMID: 33200658 Free PMC article.
-
The Phosphorylation State of the Drosophila TRP Channel Modulates the Frequency Response to Oscillating Light In Vivo.J Neurosci. 2017 Apr 12;37(15):4213-4224. doi: 10.1523/JNEUROSCI.3670-16.2017. Epub 2017 Mar 17. J Neurosci. 2017. PMID: 28314815 Free PMC article.
-
Signal-dependent hydrolysis of phosphatidylinositol 4,5-bisphosphate without activation of phospholipase C: implications on gating of Drosophila TRPL (transient receptor potential-like) channel.J Biol Chem. 2012 Jan 6;287(2):1436-47. doi: 10.1074/jbc.M111.266585. Epub 2011 Nov 7. J Biol Chem. 2012. PMID: 22065576 Free PMC article.
-
Regulation of transient receptor potential channels by the phospholipase C pathway.Adv Biol Regul. 2013 Sep;53(3):341-55. doi: 10.1016/j.jbior.2013.07.004. Epub 2013 Jul 17. Adv Biol Regul. 2013. PMID: 23916247 Free PMC article. Review.
Cited by
-
Retinal TRP channels: Cell-type-specific regulators of retinal homeostasis and multimodal integration.Prog Retin Eye Res. 2023 Jan;92:101114. doi: 10.1016/j.preteyeres.2022.101114. Epub 2022 Sep 24. Prog Retin Eye Res. 2023. PMID: 36163161 Free PMC article. Review.
-
Daily Regulation of Phototransduction, Circadian Clock, DNA Repair, and Immune Gene Expression by Heme Oxygenase in the Retina of Drosophila.Genes (Basel). 2018 Dec 21;10(1):6. doi: 10.3390/genes10010006. Genes (Basel). 2018. PMID: 30583479 Free PMC article.
-
Loss of Na(+)/K(+)-ATPase in Drosophila photoreceptors leads to blindness and age-dependent neurodegeneration.Exp Neurol. 2014 Nov;261:791-801. doi: 10.1016/j.expneurol.2014.08.025. Epub 2014 Sep 7. Exp Neurol. 2014. PMID: 25205229 Free PMC article.
-
Negative regulation of the novel norpA(P24) suppressor, diehard4, in the endo-lysosomal trafficking underlies photoreceptor cell degeneration.PLoS Genet. 2013 Jun;9(6):e1003559. doi: 10.1371/journal.pgen.1003559. Epub 2013 Jun 6. PLoS Genet. 2013. PMID: 23754968 Free PMC article.
-
PI(4,5)P2: signaling the plasma membrane.Biochem J. 2022 Nov 11;479(21):2311-2325. doi: 10.1042/BCJ20220445. Biochem J. 2022. PMID: 36367756 Free PMC article.
References
-
- Barret C., Roy C., Montcourrier P., Mangeat P., Niggli V. (2000). Mutagenesis of the phosphatidylinositol 4,5-bisphosphate (PIP(2)) binding site in the NH(2)-terminal domain of ezrin correlates with its altered cellular distribution. J. Cell Biol. 151, 1067–1080 10.1083/jcb.151.5.1067 - DOI - PMC - PubMed
-
- Berryman M., Franck Z., Bretscher A. (1993). Ezrin is concentrated in the apical microvilli of a wide variety of epithelial cells whereas moesin is found primarily in endothelial cells. J. Cell Sci. 105, 1025–1043 - PubMed
Publication types
MeSH terms
Substances
Grants and funding
LinkOut - more resources
Full Text Sources
Other Literature Sources
Molecular Biology Databases
Research Materials
Miscellaneous