Protein homeostasis as a therapeutic target for diseases of protein conformation
- PMID: 23339312
- PMCID: PMC3955168
- DOI: 10.2174/1568026611212220014
Protein homeostasis as a therapeutic target for diseases of protein conformation
Abstract
Protein misfolding and aggregation are widely implicated in an increasing number of human diseases providing for new therapeutic opportunities targeting protein homeostasis (proteostasis). The cellular response to proteotoxicity is highly regulated by stress signaling pathways, molecular chaperones, transport and clearance machineries that function as a proteostasis network (PN) to protect the stability and functional properties of the proteome. Consequently, the PN is essential at the cellular and organismal level for development and lifespan. However, when challenged during aging, stress, and disease, the folding and clearance machineries can become compromised leading to both gain-of-function and loss-of-function proteinopathies. Here, we assess the role of small molecules that activate the heat shock response, the unfolded protein response, and clearance mechanisms to increase PN capacity and protect cellular proteostasis against proteotoxicity. We propose that this strategy to enhance cell stress pathways and chaperone activity establishes a cytoprotective state against misfolding and/or aggregation and represents a promising therapeutic avenue to prevent the cellular damage associated with the variety of protein conformational diseases.
Conflict of interest statement
R.I.M. is founder, shareholder, and paid consultant for Proteostasis Therapeutics Inc. (Cambridge, MA) that is developing small molecule therapeutics for protein misfolding diseases.
Figures
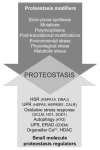
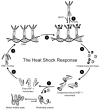
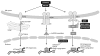
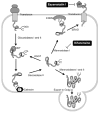
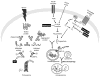
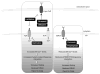
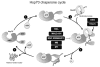
Similar articles
-
Underlying mechanisms and chemical/biochemical therapeutic approaches to ameliorate protein misfolding neurodegenerative diseases.Biofactors. 2017 Nov;43(6):737-759. doi: 10.1002/biof.1264. Epub 2016 Feb 22. Biofactors. 2017. PMID: 26899445 Review.
-
Model systems of protein-misfolding diseases reveal chaperone modifiers of proteotoxicity.Dis Model Mech. 2016 Aug 1;9(8):823-38. doi: 10.1242/dmm.024703. Dis Model Mech. 2016. PMID: 27491084 Free PMC article. Review.
-
The Chemical Biology of Molecular Chaperones--Implications for Modulation of Proteostasis.J Mol Biol. 2015 Sep 11;427(18):2931-47. doi: 10.1016/j.jmb.2015.05.010. Epub 2015 May 21. J Mol Biol. 2015. PMID: 26003923 Free PMC article. Review.
-
In vivo aspects of protein folding and quality control.Science. 2016 Jul 1;353(6294):aac4354. doi: 10.1126/science.aac4354. Science. 2016. PMID: 27365453 Review.
-
Small-molecule proteostasis regulators for protein conformational diseases.Nat Chem Biol. 2011 Dec 25;8(2):185-96. doi: 10.1038/nchembio.763. Nat Chem Biol. 2011. PMID: 22198733 Free PMC article.
Cited by
-
Sorting out the trash: the spatial nature of eukaryotic protein quality control.Curr Opin Cell Biol. 2014 Feb;26:139-146. doi: 10.1016/j.ceb.2013.12.006. Epub 2014 Jan 23. Curr Opin Cell Biol. 2014. PMID: 24463332 Free PMC article. Review.
-
The Proteome Folding Problem and Cellular Proteostasis.J Mol Biol. 2021 Oct 1;433(20):167197. doi: 10.1016/j.jmb.2021.167197. Epub 2021 Aug 13. J Mol Biol. 2021. PMID: 34391802 Free PMC article. Review.
-
Nrf2 mitigates LRRK2- and α-synuclein-induced neurodegeneration by modulating proteostasis.Proc Natl Acad Sci U S A. 2017 Jan 31;114(5):1165-1170. doi: 10.1073/pnas.1522872114. Epub 2016 Dec 27. Proc Natl Acad Sci U S A. 2017. PMID: 28028237 Free PMC article.
-
Editorial: The HSP70 Molecular Chaperone Machines.Front Mol Biosci. 2017 Jan 24;4:1. doi: 10.3389/fmolb.2017.00001. eCollection 2017. Front Mol Biosci. 2017. PMID: 28174697 Free PMC article. No abstract available.
-
Pharmaceutical Chaperones and Proteostasis Regulators in the Therapy of Lysosomal Storage Disorders: Current Perspective and Future Promises.Front Pharmacol. 2017 Jul 7;8:448. doi: 10.3389/fphar.2017.00448. eCollection 2017. Front Pharmacol. 2017. PMID: 28736525 Free PMC article. Review.
References
-
- Balch WE, Morimoto RI, Dillin A, Kelly JW. Adapting proteostasis for disease intervention. Science. 2008;319(5865):916–9. - PubMed
-
- Walter P, Ron D. The unfolded protein response: from stress pathway to homeostatic regulation. Science. 2011;334(6059):1081–6. - PubMed
-
- Powers ET, Morimoto RI, Dillin A, Kelly JW, Balch WE. Biological and chemical approaches to diseases of proteostasis deficiency. Annual review of biochemistry. 2009;78:959–91. - PubMed
-
- Gidalevitz T, Ben-Zvi A, Ho KH, Brignull HR, Morimoto RI. Progressive disruption of cellular protein folding in models of polyglutamine diseases. Science. 2006;311(5766):1471–4. - PubMed
Publication types
MeSH terms
Substances
Grants and funding
LinkOut - more resources
Full Text Sources
Other Literature Sources