The free fatty acid receptor G protein-coupled receptor 40 (GPR40) protects from bone loss through inhibition of osteoclast differentiation
- PMID: 23335512
- PMCID: PMC3585087
- DOI: 10.1074/jbc.M112.429084
The free fatty acid receptor G protein-coupled receptor 40 (GPR40) protects from bone loss through inhibition of osteoclast differentiation
Abstract
The mechanisms linking fat intake to bone loss remain unclear. By demonstrating the expression of the free fatty acid receptor G-coupled protein receptor 40 (GPR40) in bone cells, we hypothesized that this receptor may play a role in mediating the effects of fatty acids on bone remodeling. Using micro-CT analysis, we showed that GPR40(-/-) mice exhibit osteoporotic features suggesting a positive role of GPR40 on bone density. In primary cultures of bone marrow, we showed that GW9508, a GRP40 agonist, abolished bone-resorbing cell differentiation. This alteration of the receptor activator of NF-κB ligand (RANKL)-induced osteoclast differentiation occurred via the inhibition of the nuclear factor κB (NF-κB) signaling pathway as demonstrated by decrease in gene reporter activity, inhibitor of κB kinase (IKKα/β) activation, inhibitor of κB (IkBα) phosphorylation, and nuclear factor of activated T cells 1 (NFATc1) expression. The GPR40-dependent effect of GW9508 was confirmed using shRNA interference in osteoclast precursors and GPR40(-/-) primary cell cultures. In addition, in vivo administration of GW9508 counteracted ovariectomy-induced bone loss in wild-type but not GPR40(-/-) mice, enlightening the obligatory role of the GPR40 receptor. Then, in a context of growing prevalence of metabolic and age-related bone disorders, our results demonstrate for the first time in translational approaches that GPR40 is a relevant target for the design of new nutritional and therapeutic strategies to counter bone complications.
Figures
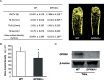
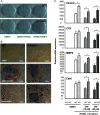
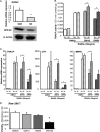
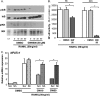
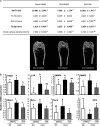
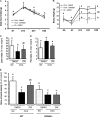
Similar articles
-
GPR40 mediates potential positive effects of a saturated fatty acid enriched diet on bone.Mol Nutr Food Res. 2017 Feb;61(2). doi: 10.1002/mnfr.201600219. Epub 2016 Nov 11. Mol Nutr Food Res. 2017. PMID: 27611773
-
Low-density lipoprotein receptor deficiency causes impaired osteoclastogenesis and increased bone mass in mice because of defect in osteoclastic cell-cell fusion.J Biol Chem. 2012 Jun 1;287(23):19229-41. doi: 10.1074/jbc.M111.323600. Epub 2012 Apr 12. J Biol Chem. 2012. PMID: 22500026 Free PMC article.
-
Caffeic acid 3,4-dihydroxy-phenethyl ester suppresses receptor activator of NF-κB ligand–induced osteoclastogenesis and prevents ovariectomy-induced bone loss through inhibition of mitogen-activated protein kinase/activator protein 1 and Ca2+–nuclear factor of activated T-cells cytoplasmic 1 signaling pathways.J Bone Miner Res. 2012 Jun;27(6):1298-1308. doi: 10.1002/jbmr.1576. J Bone Miner Res. 2012. PMID: 22337253
-
The Role of Cannabinoids in Bone Metabolism: A New Perspective for Bone Disorders.Int J Mol Sci. 2021 Nov 16;22(22):12374. doi: 10.3390/ijms222212374. Int J Mol Sci. 2021. PMID: 34830256 Free PMC article. Review.
-
The Role of Autophagy in Osteoclast Differentiation and Bone Resorption Function.Biomolecules. 2020 Sep 30;10(10):1398. doi: 10.3390/biom10101398. Biomolecules. 2020. PMID: 33008140 Free PMC article. Review.
Cited by
-
Cloning, identification and functional characterization of bovine free fatty acid receptor-1 (FFAR1/GPR40) in neutrophils.PLoS One. 2015 Mar 19;10(3):e0119715. doi: 10.1371/journal.pone.0119715. eCollection 2015. PLoS One. 2015. PMID: 25790461 Free PMC article.
-
Resveratrol reverses the negative effect of alcohol on hydroxyapatite-coated implant osseointegration in senile female rats.Z Gerontol Geriatr. 2020 Oct;53(6):538-545. doi: 10.1007/s00391-019-01595-3. Epub 2019 Aug 21. Z Gerontol Geriatr. 2020. PMID: 31435788 English.
-
G Protein-Coupled Receptors in Osteoarthritis: A Novel Perspective on Pathogenesis and Treatment.Front Cell Dev Biol. 2021 Oct 20;9:758220. doi: 10.3389/fcell.2021.758220. eCollection 2021. Front Cell Dev Biol. 2021. PMID: 34746150 Free PMC article. Review.
-
GPR120: A bi-potential mediator to modulate the osteogenic and adipogenic differentiation of BMMSCs.Sci Rep. 2015 Sep 14;5:14080. doi: 10.1038/srep14080. Sci Rep. 2015. PMID: 26365922 Free PMC article.
-
Regulation of Osteoclast Differentiation and Activity by Lipid Metabolism.Cells. 2021 Jan 7;10(1):89. doi: 10.3390/cells10010089. Cells. 2021. PMID: 33430327 Free PMC article. Review.
References
-
- Ono K., Kaneko H., Choudhary S., Pilbeam C. C., Lorenzo J. A., Akatsu T., Kugai N., Raisz L. G. (2005) Biphasic effect of prostaglandin E2 on osteoclast formation in spleen cell cultures: role of the EP2 receptor. J. Bone Miner. Res. 20, 23–29 - PubMed
-
- Laneuville O., Breuer D. K., Xu N., Huang Z. H., Gage D. A., Watson J. T., Lagarde M., DeWitt D. L., Smith W. L. (1995) Fatty acid substrate specificities of human prostaglandin-endoperoxide H synthase-1 and -2: formation of 12-hydroxy-(9Z, 13E/Z, 15Z)- octadecatrienoic acids from α-linolenic acid. J. Biol. Chem. 270, 19330–19336 - PubMed
-
- Raisz L. G., Alander C. B., Simmons H. A. (1989) Effects of prostaglandin E3 and eicosapentaenoic acid on rat bone in organ culture. Prostaglandins 37, 615–625 - PubMed
-
- Corwin R. L. (2003) Effects of dietary fats on bone health in advanced age. Prostaglandins Leukot. Essent. Fatty Acids 68, 379–386 - PubMed
Publication types
MeSH terms
Substances
Grants and funding
LinkOut - more resources
Full Text Sources
Other Literature Sources
Medical
Molecular Biology Databases
Miscellaneous