Metabolism and the circadian clock converge
- PMID: 23303907
- PMCID: PMC3781773
- DOI: 10.1152/physrev.00016.2012
Metabolism and the circadian clock converge
Abstract
Circadian rhythms occur in almost all species and control vital aspects of our physiology, from sleeping and waking to neurotransmitter secretion and cellular metabolism. Epidemiological studies from recent decades have supported a unique role for circadian rhythm in metabolism. As evidenced by individuals working night or rotating shifts, but also by rodent models of circadian arrhythmia, disruption of the circadian cycle is strongly associated with metabolic imbalance. Some genetically engineered mouse models of circadian rhythmicity are obese and show hallmark signs of the metabolic syndrome. Whether these phenotypes are due to the loss of distinct circadian clock genes within a specific tissue versus the disruption of rhythmic physiological activities (such as eating and sleeping) remains a cynosure within the fields of chronobiology and metabolism. Becoming more apparent is that from metabolites to transcription factors, the circadian clock interfaces with metabolism in numerous ways that are essential for maintaining metabolic homeostasis.
Figures
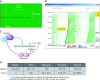
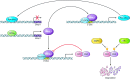
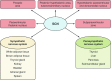
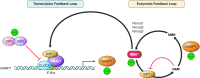
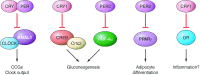
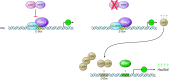
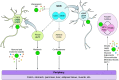
Similar articles
-
How sleep and wakefulness influence circadian rhythmicity: effects of insufficient and mistimed sleep on the animal and human transcriptome.J Sleep Res. 2015 Oct;24(5):476-93. doi: 10.1111/jsr.12307. Epub 2015 Jun 8. J Sleep Res. 2015. PMID: 26059855 Review.
-
Metabolic implications of circadian disruption.Pflugers Arch. 2020 May;472(5):513-526. doi: 10.1007/s00424-020-02381-6. Epub 2020 May 4. Pflugers Arch. 2020. PMID: 32363530 Review.
-
Circadian rhythms, sleep, and metabolism.J Clin Invest. 2011 Jun;121(6):2133-41. doi: 10.1172/JCI46043. Epub 2011 Jun 1. J Clin Invest. 2011. PMID: 21633182 Free PMC article. Review.
-
Circadian clock genes and sleep homeostasis.Eur J Neurosci. 2009 May;29(9):1820-9. doi: 10.1111/j.1460-9568.2009.06723.x. Epub 2009 Apr 28. Eur J Neurosci. 2009. PMID: 19473235 Review.
-
Regulation of metabolism: the circadian clock dictates the time.Trends Endocrinol Metab. 2012 Jan;23(1):1-8. doi: 10.1016/j.tem.2011.10.005. Epub 2011 Dec 12. Trends Endocrinol Metab. 2012. PMID: 22169754 Free PMC article. Review.
Cited by
-
Health implications of disrupted circadian rhythms and the potential for daylight as therapy.Anesthesiology. 2015 May;122(5):1170-5. doi: 10.1097/ALN.0000000000000596. Anesthesiology. 2015. PMID: 25635592 Free PMC article. Review. No abstract available.
-
The Fragile X proteins Fmrp and Fxr2p cooperate to regulate glucose metabolism in mice.Hum Mol Genet. 2015 Apr 15;24(8):2175-84. doi: 10.1093/hmg/ddu737. Epub 2014 Dec 30. Hum Mol Genet. 2015. PMID: 25552647 Free PMC article.
-
The transcriptional repressor Rev-erbα regulates circadian expression of the astrocyte Fabp7 mRNA.Curr Res Neurobiol. 2021;2:100009. doi: 10.1016/j.crneur.2021.100009. Epub 2021 Mar 23. Curr Res Neurobiol. 2021. PMID: 34056625 Free PMC article.
-
Dawn- and dusk-phased circadian transcription rhythms coordinate anabolic and catabolic functions in Neurospora.BMC Biol. 2015 Feb 24;13:17. doi: 10.1186/s12915-015-0126-4. BMC Biol. 2015. PMID: 25762222 Free PMC article.
-
Eat, Train, Sleep-Retreat? Hormonal Interactions of Intermittent Fasting, Exercise and Circadian Rhythm.Biomolecules. 2021 Mar 30;11(4):516. doi: 10.3390/biom11040516. Biomolecules. 2021. PMID: 33808424 Free PMC article. Review.
References
-
- Abrahamson EE, Moore RY. Lesions of suprachiasmatic nucleus efferents selectively affect rest-activity rhythm. Mol Cell Endocrinol 252: 46–56, 2006 - PubMed
-
- Akhtar RA, Reddy AB, Maywood ES, Clayton JD, King VM, Smith AG, Gant TW, Hastings MH, Kyriacou CP. Circadian cycling of the mouse liver transcriptome, as revealed by cDNA microarray, is driven by the suprachiasmatic nucleus. Curr Biol 12: 540–550, 2002 - PubMed
-
- Albrecht U, Zheng B, Larkin D, Sun ZS, Lee CC. MPer1 and mper2 are essential for normal resetting of the circadian clock. J Biol Rhythms 16: 100–104, 2001 - PubMed
-
- Antunes LC, Levandovski R, Dantas G, Caumo W, Hidalgo MP. Obesity and shift work: chronobiological aspects. Nutr Res Rev 23: 155–168, 2010 - PubMed
Publication types
MeSH terms
Substances
Grants and funding
LinkOut - more resources
Full Text Sources
Other Literature Sources
Molecular Biology Databases
Research Materials