Specialized role of migratory dendritic cells in peripheral tolerance induction
- PMID: 23298832
- PMCID: PMC3561796
- DOI: 10.1172/JCI65260
Specialized role of migratory dendritic cells in peripheral tolerance induction
Abstract
Harnessing DCs for immunotherapies in vivo requires the elucidation of the physiological role of distinct DC populations. Migratory DCs traffic from peripheral tissues to draining lymph nodes charged with tissue self antigens. We hypothesized that these DC populations have a specialized role in the maintenance of peripheral tolerance, specifically, to generate suppressive Foxp3+ Tregs. To examine the differential capacity of migratory DCs versus blood-derived lymphoid-resident DCs for Treg generation in vivo, we targeted a self antigen, myelin oligodendrocyte glycoprotein, using antibodies against cell surface receptors differentially expressed in these DC populations. Using this approach together with mouse models that lack specific DC populations, we found that migratory DCs have a superior ability to generate Tregs in vivo, which in turn drastically improve the outcome of experimental autoimmune encephalomyelitis. These results provide a rationale for the development of novel therapies targeting migratory DCs for the treatment of autoimmune diseases.
Figures
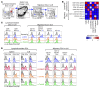
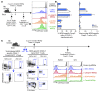
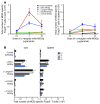
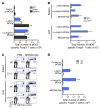
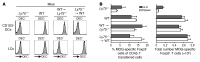
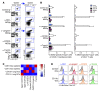

Similar articles
-
Targeting DEC-205-DCIR2+ dendritic cells promotes immunological tolerance in proteolipid protein-induced experimental autoimmune encephalomyelitis.Mol Med. 2018 May 3;24(1):17. doi: 10.1186/s10020-018-0017-6. Mol Med. 2018. PMID: 30134798 Free PMC article.
-
Steady state migratory RelB+ langerin+ dermal dendritic cells mediate peripheral induction of antigen-specific CD4+ CD25+ Foxp3+ regulatory T cells.Eur J Immunol. 2011 May;41(5):1420-34. doi: 10.1002/eji.201040930. Epub 2011 Apr 14. Eur J Immunol. 2011. PMID: 21469094
-
Comparable T helper 1 (Th1) and CD8 T-cell immunity by targeting HIV gag p24 to CD8 dendritic cells within antibodies to Langerin, DEC205, and Clec9A.Proc Natl Acad Sci U S A. 2011 Feb 8;108(6):2384-9. doi: 10.1073/pnas.1019547108. Epub 2011 Jan 24. Proc Natl Acad Sci U S A. 2011. PMID: 21262813 Free PMC article.
-
Dendritic cells and T cells in the regulation of cutaneous immunity.Adv Dermatol. 2007;23:307-33. doi: 10.1016/j.yadr.2007.07.014. Adv Dermatol. 2007. PMID: 18159907 Review.
-
Targeted antigen delivery to DEC-205⁺ dendritic cells for tolerogenic vaccination.Rev Diabet Stud. 2012 Winter;9(4):305-18. doi: 10.1900/RDS.2012.9.305. Epub 2012 Dec 28. Rev Diabet Stud. 2012. PMID: 23804268 Free PMC article. Review.
Cited by
-
Dendritic cells: cellular mediators for immunological tolerance.Clin Dev Immunol. 2013;2013:972865. doi: 10.1155/2013/972865. Epub 2013 May 15. Clin Dev Immunol. 2013. PMID: 23762100 Free PMC article. Review.
-
Dendritic cells in the periphery control antigen-specific natural and induced regulatory T cells.Front Immunol. 2013 Jun 21;4:151. doi: 10.3389/fimmu.2013.00151. eCollection 2013. Front Immunol. 2013. PMID: 23801989 Free PMC article.
-
Trial watch: Dendritic cell (DC)-based immunotherapy for cancer.Oncoimmunology. 2022 Jul 4;11(1):2096363. doi: 10.1080/2162402X.2022.2096363. eCollection 2022. Oncoimmunology. 2022. PMID: 35800158 Free PMC article.
-
An Ionizable Lipid Material with a Vitamin E Scaffold as an mRNA Vaccine Platform for Efficient Cytotoxic T Cell Responses.ACS Nano. 2023 Oct 10;17(19):18758-18774. doi: 10.1021/acsnano.3c02251. Epub 2023 Sep 26. ACS Nano. 2023. PMID: 37814788 Free PMC article.
-
Langerin+CD8+ Dendritic Cells in the Splenic Marginal Zone: Not So Marginal After All.Front Immunol. 2019 Apr 12;10:741. doi: 10.3389/fimmu.2019.00741. eCollection 2019. Front Immunol. 2019. PMID: 31031751 Free PMC article. Review.
References
Publication types
MeSH terms
Substances
Grants and funding
LinkOut - more resources
Full Text Sources
Other Literature Sources
Molecular Biology Databases