Tubulin acetyltransferase αTAT1 destabilizes microtubules independently of its acetylation activity
- PMID: 23275437
- PMCID: PMC3592022
- DOI: 10.1128/MCB.01044-12
Tubulin acetyltransferase αTAT1 destabilizes microtubules independently of its acetylation activity
Abstract
Acetylation of α-tubulin at lysine 40 (K40) is a well-conserved posttranslational modification that marks long-lived microtubules but has poorly understood functional significance. Recently, αTAT1, a member of the Gcn5-related N-acetyltransferase superfamily, has been identified as an α-tubulin acetyltransferase in ciliated organisms. Here, we explored the function of αTAT1 with the aim of understanding the consequences of αTAT1-mediated microtubule acetylation. We demonstrate that α-tubulin is the major target of αTAT1 but that αTAT1 also acetylates itself in a regulatory mechanism that is required for effective modification of tubulin. We further show that in mammalian cells, αTAT1 promotes microtubule destabilization and accelerates microtubule dynamics. Intriguingly, this effect persists in an αTAT1 mutant with no acetyltransferase activity, suggesting that interaction of αTAT1 with microtubules, rather than acetylation per se, is the critical factor regulating microtubule stability. Our data demonstrate that αTAT1 has cellular functions that extend beyond its classical enzymatic activity as an α-tubulin acetyltransferase.
Figures
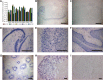
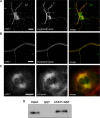
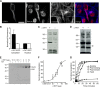
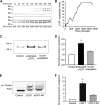
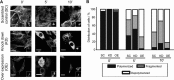
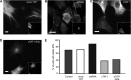
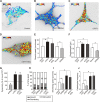
Similar articles
-
The major alpha-tubulin K40 acetyltransferase alphaTAT1 promotes rapid ciliogenesis and efficient mechanosensation.Proc Natl Acad Sci U S A. 2010 Dec 14;107(50):21517-22. doi: 10.1073/pnas.1013728107. Epub 2010 Nov 10. Proc Natl Acad Sci U S A. 2010. PMID: 21068373 Free PMC article.
-
αTAT1 is the major α-tubulin acetyltransferase in mice.Nat Commun. 2013;4:1962. doi: 10.1038/ncomms2962. Nat Commun. 2013. PMID: 23748901
-
Mechanism of microtubule lumen entry for the α-tubulin acetyltransferase enzyme αTAT1.Proc Natl Acad Sci U S A. 2016 Nov 15;113(46):E7176-E7184. doi: 10.1073/pnas.1605397113. Epub 2016 Nov 1. Proc Natl Acad Sci U S A. 2016. PMID: 27803321 Free PMC article.
-
The growing landscape of tubulin acetylation: lysine 40 and many more.Biochem J. 2016 Jul 1;473(13):1859-68. doi: 10.1042/BCJ20160172. Biochem J. 2016. PMID: 27354562 Review.
-
Tubulin acetylation: responsible enzymes, biological functions and human diseases.Cell Mol Life Sci. 2015 Nov;72(22):4237-55. doi: 10.1007/s00018-015-2000-5. Epub 2015 Jul 31. Cell Mol Life Sci. 2015. PMID: 26227334 Free PMC article. Review.
Cited by
-
Expression of α-Tubulin Acetyltransferase 1 and Tubulin Acetylation as Selective Forces in Cell Competition.Cells. 2021 Feb 14;10(2):390. doi: 10.3390/cells10020390. Cells. 2021. PMID: 33672816 Free PMC article.
-
Inhibition of HDAC6 deacetylase activity increases its binding with microtubules and suppresses microtubule dynamic instability in MCF-7 cells.J Biol Chem. 2013 Aug 2;288(31):22516-26. doi: 10.1074/jbc.M113.489328. Epub 2013 Jun 24. J Biol Chem. 2013. PMID: 23798680 Free PMC article.
-
Role of LPS-elicited signaling in triggering gastric mucosal inflammatory responses to H. pylori: modulatory effect of ghrelin.Inflammopharmacology. 2017 Aug;25(4):415-429. doi: 10.1007/s10787-017-0360-1. Epub 2017 May 17. Inflammopharmacology. 2017. PMID: 28516374 Review.
-
The α-tubulin acetyltransferase ATAT1: structure, cellular functions, and its emerging role in human diseases.Cell Mol Life Sci. 2024 Apr 23;81(1):193. doi: 10.1007/s00018-024-05227-x. Cell Mol Life Sci. 2024. PMID: 38652325 Free PMC article. Review.
-
Spatial pattern formation in microtubule post-translational modifications and the tight localization of motor-driven cargo.J Math Biol. 2017 Apr;74(5):1059-1080. doi: 10.1007/s00285-016-1053-x. Epub 2016 Sep 3. J Math Biol. 2017. PMID: 27592217 Free PMC article.
References
-
- Reed NA, Cai D, Blasius TL, Jih GT, Meyhofer E, Gaertig J, Verhey KJ. 2006. Microtubule acetylation promotes kinesin-1 binding and transport. Curr. Biol. 16: 2166–2172 - PubMed
Publication types
MeSH terms
Substances
LinkOut - more resources
Full Text Sources
Other Literature Sources
Molecular Biology Databases