Snapshots: chromatin control of viral infection
- PMID: 23217624
- PMCID: PMC3531885
- DOI: 10.1016/j.virol.2012.09.023
Snapshots: chromatin control of viral infection
Abstract
Like their cellular host counterparts, many invading viral pathogens must contend with, modulate, and utilize the host cell's chromatin machinery to promote efficient lytic infection or control persistent-latent states. While not intended to be comprehensive, this review represents a compilation of conceptual snapshots of the dynamic interplay of viruses with the chromatin environment. Contributions focus on chromatin dynamics during infection, viral circumvention of cellular chromatin repression, chromatin organization of large DNA viruses, tethering and persistence, viral interactions with cellular chromatin modulation machinery, and control of viral latency-reactivation cycles.
Published by Elsevier Inc.
Figures
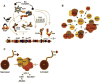
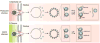
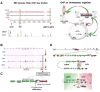
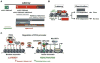
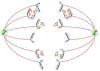
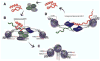
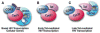
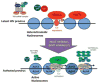
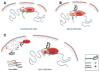
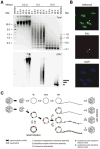
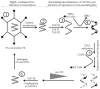
Similar articles
-
Chromatin regulation of virus infection.Trends Microbiol. 2006 Mar;14(3):132-40. doi: 10.1016/j.tim.2006.01.001. Epub 2006 Feb 2. Trends Microbiol. 2006. PMID: 16458005 Review.
-
Epigenetic and epitranscriptomic regulation of viral replication.Nat Rev Microbiol. 2020 Oct;18(10):559-570. doi: 10.1038/s41579-020-0382-3. Epub 2020 Jun 12. Nat Rev Microbiol. 2020. PMID: 32533130 Free PMC article. Review.
-
Viral Interplay with the Host Sumoylation System.Adv Exp Med Biol. 2017;963:359-388. doi: 10.1007/978-3-319-50044-7_21. Adv Exp Med Biol. 2017. PMID: 28197923 Free PMC article. Review.
-
Non-coding RNAs: novel players in chromatin-regulation during viral latency.Curr Opin Virol. 2013 Aug;3(4):387-93. doi: 10.1016/j.coviro.2013.04.001. Epub 2013 May 6. Curr Opin Virol. 2013. PMID: 23660570 Review.
-
The Interplay Between Viral-Derived miRNAs and Host Immunity During Infection.Front Immunol. 2020 Jan 23;10:3079. doi: 10.3389/fimmu.2019.03079. eCollection 2019. Front Immunol. 2020. PMID: 32038626 Free PMC article. Review.
Cited by
-
Navigating Latency-Inducing Viral Infections: Therapeutic Targeting and Nanoparticle Utilization.Biomater Res. 2024 Oct 16;28:0078. doi: 10.34133/bmr.0078. eCollection 2024. Biomater Res. 2024. PMID: 39416703 Free PMC article. Review.
-
Multifunctional Non-Coding RNAs Mediate Latent Infection and Recurrence of Herpes Simplex Viruses.Infect Drug Resist. 2021 Dec 14;14:5335-5349. doi: 10.2147/IDR.S334769. eCollection 2021. Infect Drug Resist. 2021. PMID: 34934329 Free PMC article. Review.
-
Navigating the Host Cell Response during Entry into Sites of Latent Cytomegalovirus Infection.Pathogens. 2018 Mar 16;7(1):30. doi: 10.3390/pathogens7010030. Pathogens. 2018. PMID: 29547547 Free PMC article. Review.
-
Lysine-specific post-translational modifications of proteins in the life cycle of viruses.Cell Cycle. 2019 Sep;18(17):1995-2005. doi: 10.1080/15384101.2019.1639305. Epub 2019 Jul 10. Cell Cycle. 2019. PMID: 31291816 Free PMC article. Review.
-
CCCTC-Binding Factor Acts as a Heterochromatin Barrier on Herpes Simplex Viral Latent Chromatin and Contributes to Poised Latent Infection.mBio. 2018 Feb 6;9(1):e02372-17. doi: 10.1128/mBio.02372-17. mBio. 2018. PMID: 29437926 Free PMC article.
References
-
- Alfonso R, Lutz T, Rodriguez A, Chavez JP, Rodriguez P, Gutierrez S, Nieto A. CHD6 chromatin remodeler is a negative modulator of influenza virus replication that relocates to inactive chromatin upon infection. Cell Microbiol. 2011;13:1894–1906. - PubMed
-
- Archin NM, Liberty AL, Kashuba AD, Choudhary SK, Kuruc JD, Crooks AM, Parker DC, Anderson EM, Kearney MF, Strain MC, Richman DD, Hudgens MG, Bosch RJ, Coffin JM, Eron JJ, Hazuda DJ, Margolis DM. Administration of vorinostat disrupts HIV-1 latency in patients on antiretroviral therapy. Nature. 2012;487:482–485. - PMC - PubMed
Publication types
MeSH terms
Substances
Grants and funding
- R01 DE017336/DE/NIDCR NIH HHS/United States
- R01CA093606/CA/NCI NIH HHS/United States
- P01AI099783-01/AI/NIAID NIH HHS/United States
- 5-31536/PHS HHS/United States
- R21 AI105909/AI/NIAID NIH HHS/United States
- R01 DE023926/DE/NIDCR NIH HHS/United States
- R56AI096861-01/AI/NIAID NIH HHS/United States
- R01 CA115284/CA/NCI NIH HHS/United States
- R01CA085678/CA/NCI NIH HHS/United States
- R56 AI096861/AI/NIAID NIH HHS/United States
- AI083139/AI/NIAID NIH HHS/United States
- U19 AI096113/AI/NIAID NIH HHS/United States
- R01 AI099081/AI/NIAID NIH HHS/United States
- P01 DE019085/DE/NIDCR NIH HHS/United States
- AI063106/AI/NIAID NIH HHS/United States
- R01 CA117830/CA/NCI NIH HHS/United States
- R01 CA093606/CA/NCI NIH HHS/United States
- P01 AI099783/AI/NIAID NIH HHS/United States
- ZIA AI000711-19/Intramural NIH HHS/United States
- R01 AI063106/AI/NIAID NIH HHS/United States
- R01 DA030156/DA/NIDA NIH HHS/United States
- CAPMC/ CIHR/Canada
- ZIA AI000712-19/Intramural NIH HHS/United States
- P30 AI027763/AI/NIAID NIH HHS/United States
- R01DE017336/DE/NIDCR NIH HHS/United States
- P01DE019085/DE/NIDCR NIH HHS/United States
- R01CA117830/CA/NCI NIH HHS/United States
- R01 CA085678/CA/NCI NIH HHS/United States
- AI099081/AI/NIAID NIH HHS/United States
LinkOut - more resources
Full Text Sources
Other Literature Sources
Medical