Perspectives on: conformational coupling in ion channels: thermodynamics of electromechanical coupling in voltage-gated ion channels
- PMID: 23183697
- PMCID: PMC3514737
- DOI: 10.1085/jgp.201210840
Perspectives on: conformational coupling in ion channels: thermodynamics of electromechanical coupling in voltage-gated ion channels
Figures
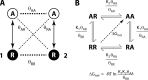
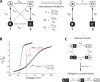
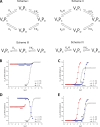
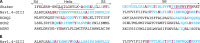
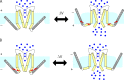
Similar articles
-
Structure and Sequence-based Computational Approaches to Allosteric Signal Transduction: Application to Electromechanical Coupling in Voltage-gated Ion Channels.J Mol Biol. 2021 Aug 20;433(17):167095. doi: 10.1016/j.jmb.2021.167095. Epub 2021 Jun 6. J Mol Biol. 2021. PMID: 34107281 Review.
-
Structure and function of voltage-gated ion channels.Naturwissenschaften. 1998 Sep;85(9):437-44. doi: 10.1007/s001140050527. Naturwissenschaften. 1998. PMID: 9802045 Review.
-
Perspectives on: conformational coupling in ion channels.J Gen Physiol. 2012 Dec;140(6):595-7. doi: 10.1085/jgp.201210926. J Gen Physiol. 2012. PMID: 23183695 Free PMC article. No abstract available.
-
Mapping Electromechanical Coupling Pathways in Voltage-Gated Ion Channels: Challenges and the Way Forward.J Mol Biol. 2021 Aug 20;433(17):167104. doi: 10.1016/j.jmb.2021.167104. Epub 2021 Jun 15. J Mol Biol. 2021. PMID: 34139217 Free PMC article. Review.
-
A charged view of voltage-gated ion channels.Nat Struct Biol. 2003 Jun;10(6):422-4. doi: 10.1038/nsb0603-422. Nat Struct Biol. 2003. PMID: 12768203 No abstract available.
Cited by
-
A charged residue in S4 regulates coupling among the activation gate, voltage, and Ca2+ sensors in BK channels.J Neurosci. 2014 Sep 10;34(37):12280-8. doi: 10.1523/JNEUROSCI.1174-14.2014. J Neurosci. 2014. PMID: 25209270 Free PMC article.
-
Interfacial gating triad is crucial for electromechanical transduction in voltage-activated potassium channels.J Gen Physiol. 2014 Nov;144(5):457-67. doi: 10.1085/jgp.201411185. Epub 2014 Oct 13. J Gen Physiol. 2014. PMID: 25311635 Free PMC article.
-
A self-consistent approach for determining pairwise interactions that underlie channel activation.J Gen Physiol. 2014 Nov;144(5):441-55. doi: 10.1085/jgp.201411184. Epub 2014 Oct 13. J Gen Physiol. 2014. PMID: 25311637 Free PMC article.
-
Perspectives on: conformational coupling in ion channels: conformational coupling in BK potassium channels.J Gen Physiol. 2012 Dec;140(6):625-34. doi: 10.1085/jgp.201210849. J Gen Physiol. 2012. PMID: 23183698 Free PMC article.
-
A PIP2 substitute mediates voltage sensor-pore coupling in KCNQ activation.Commun Biol. 2020 Jul 16;3(1):385. doi: 10.1038/s42003-020-1104-0. Commun Biol. 2020. PMID: 32678288 Free PMC article.
References
Publication types
MeSH terms
Substances
Grants and funding
LinkOut - more resources
Full Text Sources