Function and regulation of SUMO proteases
- PMID: 23175280
- PMCID: PMC3668692
- DOI: 10.1038/nrm3478
Function and regulation of SUMO proteases
Abstract
Covalent attachment of small ubiquitin-like modifier (SUMO) to proteins is highly dynamic, and both SUMO-protein conjugation and cleavage can be regulated. Protein desumoylation is carried out by SUMO proteases, which control cellular mechanisms ranging from transcription and cell division to ribosome biogenesis. Recent advances include the discovery of two novel classes of SUMO proteases, insights regarding SUMO protease specificity, and revelations of previously unappreciated SUMO protease functions in several key cellular pathways. These developments, together with new connections between SUMO proteases and the recently discovered SUMO-targeted ubiquitin ligases (STUbLs), make this an exciting period to study these enzymes.
Figures
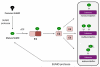
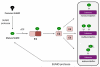
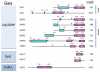
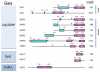
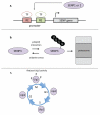
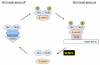
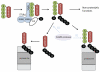
Similar articles
-
A global S. cerevisiae small ubiquitin-related modifier (SUMO) system interactome.Mol Syst Biol. 2013 May 28;9:668. doi: 10.1038/msb.2013.23. Mol Syst Biol. 2013. PMID: 23712011 Free PMC article.
-
Recruitment of a SUMO isopeptidase to rDNA stabilizes silencing complexes by opposing SUMO targeted ubiquitin ligase activity.Genes Dev. 2017 Apr 15;31(8):802-815. doi: 10.1101/gad.296145.117. Epub 2017 May 9. Genes Dev. 2017. PMID: 28487408 Free PMC article.
-
SUMO-independent in vivo activity of a SUMO-targeted ubiquitin ligase toward a short-lived transcription factor.Genes Dev. 2010 May;24(9):893-903. doi: 10.1101/gad.1906510. Epub 2010 Apr 13. Genes Dev. 2010. PMID: 20388728 Free PMC article.
-
SUMO-specific proteases: a twist in the tail.Trends Cell Biol. 2007 Aug;17(8):370-6. doi: 10.1016/j.tcb.2007.08.002. Epub 2007 Sep 4. Trends Cell Biol. 2007. PMID: 17768054 Review.
-
Genome maintenance in Saccharomyces cerevisiae: the role of SUMO and SUMO-targeted ubiquitin ligases.Nucleic Acids Res. 2017 Mar 17;45(5):2242-2261. doi: 10.1093/nar/gkw1369. Nucleic Acids Res. 2017. PMID: 28115630 Free PMC article. Review.
Cited by
-
Cellular SUMO-specific proteases regulate HAdV-C5 E1B-55K SUMOylation and virus-induced cell transformation.Front Cell Infect Microbiol. 2024 Sep 27;14:1484241. doi: 10.3389/fcimb.2024.1484241. eCollection 2024. Front Cell Infect Microbiol. 2024. PMID: 39397864 Free PMC article.
-
SUMO-Specific Protease 2 (SENP2) Is an Important Regulator of Fatty Acid Metabolism in Skeletal Muscle.Diabetes. 2015 Jul;64(7):2420-31. doi: 10.2337/db15-0115. Epub 2015 Mar 17. Diabetes. 2015. PMID: 25784542 Free PMC article.
-
SUMOylation regulates Lem2 function in centromere clustering and silencing.J Cell Sci. 2023 Dec 1;136(23):jcs260868. doi: 10.1242/jcs.260868. Epub 2023 Dec 1. J Cell Sci. 2023. PMID: 37970674 Free PMC article.
-
Revised annotation and extended characterizations of components of the Chlamydomonas reinhardtii SUMOylation system.Plant Direct. 2020 Sep 28;4(9):e00266. doi: 10.1002/pld3.266. eCollection 2020 Sep. Plant Direct. 2020. PMID: 33015534 Free PMC article.
-
New insight into a simple high-yielding method for the production of fully folded and functional recombinant human CCL5.Sci Rep. 2024 Oct 15;14(1):24188. doi: 10.1038/s41598-024-75327-y. Sci Rep. 2024. PMID: 39406925 Free PMC article.
References
-
- Panse VG, Hardeland U, Werner T, Kuster B, Hurt E. A proteome-wide approach identifies sumoylated substrate proteins in yeast. J Biol Chem. 2004;279:41346–51. - PubMed
-
- Wohlschlegel JA, Johnson ES, Reed SI, Yates JR., 3rd Global analysis of protein sumoylation in Saccharomyces cerevisiae. J Biol Chem. 2004;279:45662–8. - PubMed
-
- Hannich JT, et al. Defining the SUMO-modified proteome by multiple approaches in Saccharomyces cerevisiae. J Biol Chem. 2005;280:4102–10. - PubMed
-
- Wykoff DD, O'Shea EK. Identification of sumoylated proteins by systematic immunoprecipitation of the budding yeast proteome. Mol Cell Proteomics. 2005;4:73–83. - PubMed
Publication types
MeSH terms
Substances
Grants and funding
LinkOut - more resources
Full Text Sources
Other Literature Sources
Molecular Biology Databases