Controls of nucleosome positioning in the human genome
- PMID: 23166509
- PMCID: PMC3499251
- DOI: 10.1371/journal.pgen.1003036
Controls of nucleosome positioning in the human genome
Abstract
Nucleosomes are important for gene regulation because their arrangement on the genome can control which proteins bind to DNA. Currently, few human nucleosomes are thought to be consistently positioned across cells; however, this has been difficult to assess due to the limited resolution of existing data. We performed paired-end sequencing of micrococcal nuclease-digested chromatin (MNase-seq) from seven lymphoblastoid cell lines and mapped over 3.6 billion MNase-seq fragments to the human genome to create the highest-resolution map of nucleosome occupancy to date in a human cell type. In contrast to previous results, we find that most nucleosomes have more consistent positioning than expected by chance and a substantial fraction (8.7%) of nucleosomes have moderate to strong positioning. In aggregate, nucleosome sequences have 10 bp periodic patterns in dinucleotide frequency and DNase I sensitivity; and, across cells, nucleosomes frequently have translational offsets that are multiples of 10 bp. We estimate that almost half of the genome contains regularly spaced arrays of nucleosomes, which are enriched in active chromatin domains. Single nucleotide polymorphisms that reduce DNase I sensitivity can disrupt the phasing of nucleosome arrays, which indicates that they often result from positioning against a barrier formed by other proteins. However, nucleosome arrays can also be created by DNA sequence alone. The most striking example is an array of over 400 nucleosomes on chromosome 12 that is created by tandem repetition of sequences with strong positioning properties. In summary, a large fraction of nucleosomes are consistently positioned--in some regions because they adopt favored sequence positions, and in other regions because they are forced into specific arrangements by chromatin remodeling or DNA binding proteins.
Conflict of interest statement
The authors have declared that no competing interests exist.
Figures
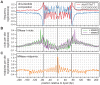
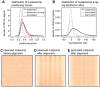
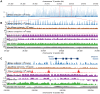
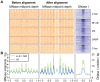
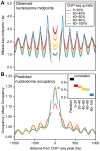
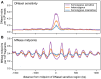
Similar articles
-
Principles of nucleosome organization revealed by single-cell micrococcal nuclease sequencing.Nature. 2018 Oct;562(7726):281-285. doi: 10.1038/s41586-018-0567-3. Epub 2018 Sep 26. Nature. 2018. PMID: 30258225 Free PMC article.
-
Subtracting the sequence bias from partially digested MNase-seq data reveals a general contribution of TFIIS to nucleosome positioning.Epigenetics Chromatin. 2017 Dec 7;10(1):58. doi: 10.1186/s13072-017-0165-x. Epigenetics Chromatin. 2017. PMID: 29212533 Free PMC article.
-
Chromatin structure of the yeast URA3 gene at high resolution provides insight into structure and positioning of nucleosomes in the chromosomal context.J Mol Biol. 1996 Apr 19;257(5):919-34. doi: 10.1006/jmbi.1996.0212. J Mol Biol. 1996. PMID: 8632475
-
Resolving nucleosomal positioning and occupancy with MNase-seq.Yi Chuan. 2020 Dec 17;42(12):1143-1155. doi: 10.16288/j.yczz.20-178. Yi Chuan. 2020. PMID: 33509779 Review.
-
Nucleosome positioning in yeasts: methods, maps, and mechanisms.Chromosoma. 2015 Jun;124(2):131-51. doi: 10.1007/s00412-014-0501-x. Epub 2014 Dec 23. Chromosoma. 2015. PMID: 25529773 Review.
Cited by
-
DFF-ChIP: a method to detect and quantify complex interactions between RNA polymerase II, transcription factors, and chromatin.Nucleic Acids Res. 2024 Oct 14;52(18):e88. doi: 10.1093/nar/gkae760. Nucleic Acids Res. 2024. PMID: 39248105 Free PMC article.
-
Effects of size, cooperativity, and competitive binding on protein positioning on DNA.Biophys J. 2021 May 18;120(10):2040-2053. doi: 10.1016/j.bpj.2021.03.016. Epub 2021 Mar 23. Biophys J. 2021. PMID: 33771470 Free PMC article.
-
CoRE-ATAC: A deep learning model for the functional classification of regulatory elements from single cell and bulk ATAC-seq data.PLoS Comput Biol. 2021 Dec 13;17(12):e1009670. doi: 10.1371/journal.pcbi.1009670. eCollection 2021 Dec. PLoS Comput Biol. 2021. PMID: 34898596 Free PMC article.
-
MNase Profiling of Promoter Chromatin in Salmonella typhimurium-Stimulated GM12878 Cells Reveals Dynamic and Response-Specific Nucleosome Architecture.G3 (Bethesda). 2020 Jul 7;10(7):2171-2178. doi: 10.1534/g3.120.401266. G3 (Bethesda). 2020. PMID: 32404364 Free PMC article.
-
The spring-loaded genome: nucleosome redistributions are widespread, transient, and DNA-directed.Genome Res. 2014 Feb;24(2):251-9. doi: 10.1101/gr.160150.113. Epub 2013 Dec 5. Genome Res. 2014. PMID: 24310001 Free PMC article.
References
-
- Kornberg RD (1974) Chromatin structure: a repeating unit of histones and DNA. Science 184: 868–871. - PubMed
-
- Kornberg RD, Lorch Y (1999) Twenty-five years of the nucleosome, fundamental particle of the eukaryote chromosome. Cell 98: 285–294. - PubMed
-
- Kaplan T, Li XY, Sabo PJ, Thomas S, Stamatoyannopoulos JA, et al. (2011) Quantitative Models of the Mechanisms That Control Genome-Wide Patterns of Transcription Factor Binding during Early Drosophila Development. PLoS Genet 7: e1001290 doi:10.1371/journal.pgen.1001290. - DOI - PMC - PubMed
-
- Albert I, Mavrich TN, Tomsho LP, Qi J, Zanton SJ, et al. (2007) Translational and rotational settings of H2A.Z nucleosomes across the Saccharomyces cerevisiae genome. Nature 446: 572–576. - PubMed
Publication types
MeSH terms
Substances
Associated data
- Actions
Grants and funding
LinkOut - more resources
Full Text Sources
Other Literature Sources
Molecular Biology Databases