Presynaptic α4β2 nicotinic acetylcholine receptors increase glutamate release and serotonin neuron excitability in the dorsal raphe nucleus
- PMID: 23100436
- PMCID: PMC6704822
- DOI: 10.1523/JNEUROSCI.0941-12.2012
Presynaptic α4β2 nicotinic acetylcholine receptors increase glutamate release and serotonin neuron excitability in the dorsal raphe nucleus
Abstract
Several behavioral effects of nicotine are mediated by changes in serotonin (5-HT) release in brain areas that receive serotonergic afferents from the dorsal raphe nucleus (DRN). In vitro experiments have demonstrated that nicotine increases the firing activity in the majority of DRN 5-HT neurons and that DRN contains nicotinic acetylcholine receptors (nAChRs) located at both somata and presynaptic elements. One of the most common presynaptic effects of nicotine is to increase glutamate release. Although DRN receives profuse glutamatergic afferents, the effect of nicotine on glutamate release in the DRN has not been studied in detail. Using whole-cell recording techniques, we investigated the effects of nicotine on the glutamatergic input to 5-HT DRN neurons in rat midbrain slices. Low nicotine concentrations, in the presence of bicuculline and tetrodotoxin (TTX), increased the frequency but did not change the amplitude of glutamate-induced EPSCs, recorded from identified 5-HT neurons. Nicotine-induced increase of glutamatergic EPSC frequency persisted 10-20 min after drug withdrawal. This nicotinic effect was mimicked by exogenous administration of acetylcholine (ACh) or inhibition of ACh metabolism. In addition, the nicotine-induced increase in EPSC frequency was abolished by blockade of α4β2 nAChRs, voltage-gated calcium channels, or intracellular calcium signaling but not by α7 nAChR antagonists. These data suggest that both nicotine and endogenous ACh can increase glutamate release through activation of presynaptic α4β2 but not α7 nAChRs in the DRN. The effect involves long-term changes in synaptic function, and it is dependent on voltage-gated calcium channels and presynaptic calcium stores.
Figures
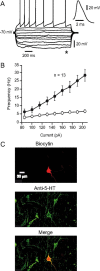
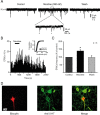
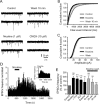
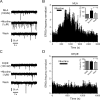
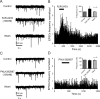
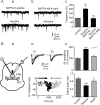
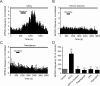
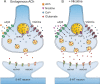
Similar articles
-
Nicotine increases GABAergic input on rat dorsal raphe serotonergic neurons through alpha7 nicotinic acetylcholine receptor.J Neurophysiol. 2014 Dec 15;112(12):3154-63. doi: 10.1152/jn.00223.2014. Epub 2014 Sep 17. J Neurophysiol. 2014. PMID: 25231613
-
Flattening plasma corticosterone levels increases the prevalence of serotonergic dorsal raphe neurons inhibitory responses to nicotine in adrenalectomised rats.Brain Res Bull. 2013 Sep;98:10-22. doi: 10.1016/j.brainresbull.2013.07.006. Epub 2013 Jul 17. Brain Res Bull. 2013. PMID: 23872451
-
Nicotine recruits a local glutamatergic circuit to excite septohippocampal GABAergic neurons.Eur J Neurosci. 2003 Sep;18(5):1155-68. doi: 10.1046/j.1460-9568.2003.02847.x. Eur J Neurosci. 2003. PMID: 12956714
-
Nicotinic modulation of serotonergic activity in the dorsal raphe nucleus.Rev Neurosci. 2013;24(5):455-69. doi: 10.1515/revneuro-2013-0012. Rev Neurosci. 2013. PMID: 24021594 Review.
-
Glutamatergic drive of the dorsal raphe nucleus.J Chem Neuroanat. 2011 Jul;41(4):247-55. doi: 10.1016/j.jchemneu.2011.04.004. Epub 2011 Apr 27. J Chem Neuroanat. 2011. PMID: 21550397 Free PMC article. Review.
Cited by
-
Molecules and circuits involved in nicotine addiction: The many faces of smoking.Neuropharmacology. 2014 Jan;76 Pt B(0 0):545-53. doi: 10.1016/j.neuropharm.2013.04.028. Epub 2013 Apr 28. Neuropharmacology. 2014. PMID: 23632083 Free PMC article. Review.
-
Is the Antidepressant Activity of Selective Serotonin Reuptake Inhibitors Mediated by Nicotinic Acetylcholine Receptors?Molecules. 2021 Apr 8;26(8):2149. doi: 10.3390/molecules26082149. Molecules. 2021. PMID: 33917953 Free PMC article. Review.
-
Additive interaction between scopolamine and nitric oxide agents on immobility in the forced swim test but not exploratory activity in the hole-board.Psychopharmacology (Berl). 2019 Nov;236(11):3353-3362. doi: 10.1007/s00213-019-05294-0. Epub 2019 Jun 7. Psychopharmacology (Berl). 2019. PMID: 31175384
-
Nicotine Increases Spontaneous Glutamate Release in the Rostromedial Tegmental Nucleus.Front Neurosci. 2021 Jan 13;14:604583. doi: 10.3389/fnins.2020.604583. eCollection 2020. Front Neurosci. 2021. PMID: 33519359 Free PMC article.
-
Possible contributions of a novel form of synaptic plasticity in Aplysia to reward, memory, and their dysfunctions in mammalian brain.Learn Mem. 2013 Sep 18;20(10):580-91. doi: 10.1101/lm.031237.113. Learn Mem. 2013. PMID: 24049187 Free PMC article. Review.
References
-
- Alkondon M, Albuquerque EX. Diversity of nicotinic acetylcholine receptors in rat hippocampal neurons. III. Agonist actions of the novel alkaloid epibatidine and analysis of type II current. J Pharmacol Exp Ther. 1995;274:771–782. - PubMed
-
- Amat J, Baratta MV, Paul E, Bland ST, Watkins LR, Maier SF. Medial prefrontal cortex determines how stressor controllability affects behavior and dorsal raphe nucleus. Nat Neurosci. 2005;8:365–371. - PubMed
-
- Bayliss DA, Li YW, Talley EM. Effects of serotonin on caudal raphe neurons: inhibition of N and P/Q-type calcium channels and the afterhyperpolarization. J Neurophysiol. 1997;77:1362–1374. - PubMed
-
- Bitner RS, Nikkel AL. Alpha-7 nicotinic receptor expression by two distinct cell types in the dorsal raphe nucleus and locus coeruleus of rat. Brain Res. 2002;938:45–54. - PubMed
Publication types
MeSH terms
Substances
LinkOut - more resources
Full Text Sources
Miscellaneous