Herpes simplex virus membrane proteins gE/gI and US9 act cooperatively to promote transport of capsids and glycoproteins from neuron cell bodies into initial axon segments
- PMID: 23077321
- PMCID: PMC3536398
- DOI: 10.1128/JVI.02465-12
Herpes simplex virus membrane proteins gE/gI and US9 act cooperatively to promote transport of capsids and glycoproteins from neuron cell bodies into initial axon segments
Abstract
Herpes simplex virus (HSV) and other alphaherpesviruses must move from sites of latency in ganglia to peripheral epithelial cells. How HSV navigates in neuronal axons is not well understood. Two HSV membrane proteins, gE/gI and US9, are key to understanding the processes by which viral glycoproteins, unenveloped capsids, and enveloped virions are transported toward axon tips. Whether gE/gI and US9 function to promote the loading of viral proteins onto microtubule motors in neuron cell bodies or to tether viral proteins onto microtubule motors within axons is not clear. One impediment to understanding how HSV gE/gI and US9 function in axonal transport relates to observations that gE(-), gI(-), or US9(-) mutants are not absolutely blocked in axonal transport. Mutants are significantly reduced in numbers of capsids and glycoproteins in distal axons, but there are less extensive effects in proximal axons. We constructed HSV recombinants lacking both gE and US9 that transported no detectable capsids and glycoproteins to distal axons and failed to spread from axon tips to adjacent cells. Live-cell imaging of a gE(-)/US9(-) double mutant that expressed fluorescent capsids and gB demonstrated >90% diminished capsids and gB in medial axons and no evidence for decreased rates of transport, stalling, or increased retrograde transport. Instead, capsids, gB, and enveloped virions failed to enter proximal axons. We concluded that gE/gI and US9 function in neuron cell bodies, in a cooperative fashion, to promote the loading of HSV capsids and vesicles containing glycoproteins and enveloped virions onto microtubule motors or their transport into proximal axons.
Figures
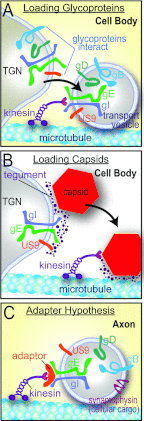
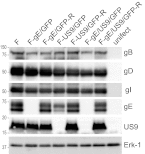

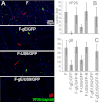
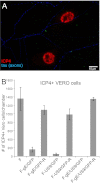
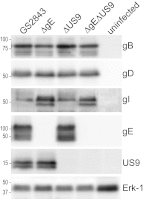
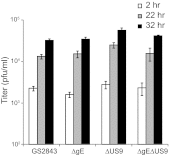
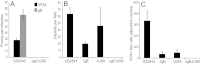

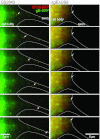
Similar articles
-
Herpes Simplex Virus gE/gI and US9 Promote both Envelopment and Sorting of Virus Particles in the Cytoplasm of Neurons, Two Processes That Precede Anterograde Transport in Axons.J Virol. 2017 May 12;91(11):e00050-17. doi: 10.1128/JVI.00050-17. Print 2017 Jun 1. J Virol. 2017. PMID: 28331094 Free PMC article.
-
Herpes simplex virus gE/gI and US9 proteins promote transport of both capsids and virion glycoproteins in neuronal axons.J Virol. 2008 Nov;82(21):10613-24. doi: 10.1128/JVI.01241-08. Epub 2008 Aug 27. J Virol. 2008. PMID: 18753205 Free PMC article.
-
Characterization of the Herpes Simplex Virus (HSV) Tegument Proteins That Bind to gE/gI and US9, Which Promote Assembly of HSV and Transport into Neuronal Axons.J Virol. 2020 Nov 9;94(23):e01113-20. doi: 10.1128/JVI.01113-20. Print 2020 Nov 9. J Virol. 2020. PMID: 32938770 Free PMC article.
-
Transport and egress of herpes simplex virus in neurons.Rev Med Virol. 2008 Jan-Feb;18(1):35-51. doi: 10.1002/rmv.560. Rev Med Virol. 2008. PMID: 17992661 Review.
-
Anterograde transport of α-herpesviruses in neuronal axons.Virology. 2021 Jul;559:65-73. doi: 10.1016/j.virol.2021.02.011. Epub 2021 Mar 4. Virology. 2021. PMID: 33836340 Review.
Cited by
-
Cell entry mechanisms of HSV: what we have learned in recent years.Future Virol. 2015 Oct 1;10(10):1145-1154. doi: 10.2217/fvl.15.85. Future Virol. 2015. PMID: 27066105 Free PMC article.
-
Keeping it in check: chronic viral infection and antiviral immunity in the brain.Nat Rev Neurosci. 2016 Dec;17(12):766-776. doi: 10.1038/nrn.2016.140. Epub 2016 Nov 4. Nat Rev Neurosci. 2016. PMID: 27811921 Free PMC article. Review.
-
Discovery and Characterization of an Aberrant Small Form of Glycoprotein I of Herpes Simplex Virus Type I in Cell Culture.Microbiol Spectr. 2022 Apr 27;10(2):e0265921. doi: 10.1128/spectrum.02659-21. Epub 2022 Mar 29. Microbiol Spectr. 2022. PMID: 35348373 Free PMC article.
-
Upsetting the Balance: When Viruses Manipulate Cell Polarity Control.J Mol Biol. 2018 Sep 28;430(19):3481-3503. doi: 10.1016/j.jmb.2018.04.016. Epub 2018 Apr 20. J Mol Biol. 2018. PMID: 29680664 Free PMC article. Review.
-
Kinesin-1 Proteins KIF5A, -5B, and -5C Promote Anterograde Transport of Herpes Simplex Virus Enveloped Virions in Axons.J Virol. 2018 Sep 26;92(20):e01269-18. doi: 10.1128/JVI.01269-18. Print 2018 Oct 15. J Virol. 2018. PMID: 30068641 Free PMC article.
References
Publication types
MeSH terms
Substances
Grants and funding
LinkOut - more resources
Full Text Sources