RUNX1 reshapes the epigenetic landscape at the onset of haematopoiesis
- PMID: 23064151
- PMCID: PMC3501222
- DOI: 10.1038/emboj.2012.275
RUNX1 reshapes the epigenetic landscape at the onset of haematopoiesis
Abstract
Cell fate decisions during haematopoiesis are governed by lineage-specific transcription factors, such as RUNX1, SCL/TAL1, FLI1 and C/EBP family members. To gain insight into how these transcription factors regulate the activation of haematopoietic genes during embryonic development, we measured the genome-wide dynamics of transcription factor assembly on their target genes during the RUNX1-dependent transition from haemogenic endothelium (HE) to haematopoietic progenitors. Using a Runx1-/- embryonic stem cell differentiation model expressing an inducible Runx1 gene, we show that in the absence of RUNX1, haematopoietic genes bind SCL/TAL1, FLI1 and C/EBPβ and that this early priming is required for correct temporal expression of the myeloid master regulator PU.1 and its downstream targets. After induction, RUNX1 binds to numerous de novo sites, initiating a local increase in histone acetylation and rapid global alterations in the binding patterns of SCL/TAL1 and FLI1. The acquisition of haematopoietic fate controlled by Runx1 therefore does not represent the establishment of a new regulatory layer on top of a pre-existing HE program but instead entails global reorganization of lineage-specific transcription factor assemblies.
Conflict of interest statement
The authors declare that they have no conflict of interest.
Figures
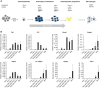
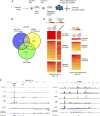
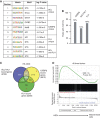
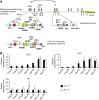
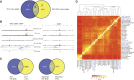
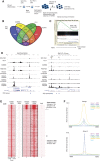
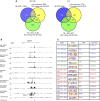
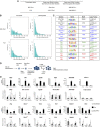
Similar articles
-
The Co-operation of RUNX1 with LDB1, CDK9 and BRD4 Drives Transcription Factor Complex Relocation During Haematopoietic Specification.Sci Rep. 2018 Jul 10;8(1):10410. doi: 10.1038/s41598-018-28506-7. Sci Rep. 2018. PMID: 29991720 Free PMC article.
-
Differential genomic targeting of the transcription factor TAL1 in alternate haematopoietic lineages.EMBO J. 2011 Feb 2;30(3):494-509. doi: 10.1038/emboj.2010.342. Epub 2010 Dec 21. EMBO J. 2011. PMID: 21179004 Free PMC article.
-
SCL/TAL1 in Hematopoiesis and Cellular Reprogramming.Curr Top Dev Biol. 2016;118:163-204. doi: 10.1016/bs.ctdb.2016.01.004. Epub 2016 Feb 18. Curr Top Dev Biol. 2016. PMID: 27137657 Review.
-
HoxA3 is an apical regulator of haemogenic endothelium.Nat Cell Biol. 2011 Jan;13(1):72-8. doi: 10.1038/ncb2137. Epub 2010 Dec 19. Nat Cell Biol. 2011. PMID: 21170035 Free PMC article.
-
Taking the Leap: Runx1 in the Formation of Blood from Endothelium.Curr Top Dev Biol. 2016;118:113-62. doi: 10.1016/bs.ctdb.2016.01.008. Epub 2016 Feb 18. Curr Top Dev Biol. 2016. PMID: 27137656 Review.
Cited by
-
Epigenetic mechanisms and developmental choice hierarchies in T-lymphocyte development.Brief Funct Genomics. 2013 Nov;12(6):512-24. doi: 10.1093/bfgp/elt027. Epub 2013 Aug 6. Brief Funct Genomics. 2013. PMID: 23922132 Free PMC article. Review.
-
Blood stem cell-forming haemogenic endothelium in zebrafish derives from arterial endothelium.Nat Commun. 2019 Aug 8;10(1):3577. doi: 10.1038/s41467-019-11423-2. Nat Commun. 2019. PMID: 31395869 Free PMC article.
-
RUNX1 maintains the identity of the fetal ovary through an interplay with FOXL2.Nat Commun. 2019 Nov 11;10(1):5116. doi: 10.1038/s41467-019-13060-1. Nat Commun. 2019. PMID: 31712577 Free PMC article.
-
Distinct temporal requirements for Runx1 in hematopoietic progenitors and stem cells.Development. 2013 Sep;140(18):3765-76. doi: 10.1242/dev.094961. Epub 2013 Aug 7. Development. 2013. PMID: 23924635 Free PMC article.
-
MAPK p38alpha Kinase Influences Haematopoiesis in Embryonic Stem Cells.Stem Cells Int. 2019 Jun 2;2019:5128135. doi: 10.1155/2019/5128135. eCollection 2019. Stem Cells Int. 2019. PMID: 31281375 Free PMC article.
References
-
- Bereshchenko O, Mancini E, Moore S, Bilbao D, Mansson R, Luc S, Grover A, Jacobsen SE, Bryder D, Nerlov C (2009) Hematopoietic stem cell expansion precedes the generation of committed myeloid leukemia-initiating cells in C/EBPalpha mutant AML. Cancer Cell 16: 390–400 - PubMed
Publication types
MeSH terms
Substances
Grants and funding
- MC_PC_12009/MRC_/Medical Research Council/United Kingdom
- 079249/WT_/Wellcome Trust/United Kingdom
- R01 CA118316/CA/NCI NIH HHS/United States
- G0901579/MRC_/Medical Research Council/United Kingdom
- G0900729/1/NC3RS_/National Centre for the Replacement, Refinement and Reduction of Animals in Research/United Kingdom
- 12765/CRUK_/Cancer Research UK/United Kingdom
- 12487/CRUK_/Cancer Research UK/United Kingdom
- 12486/CRUK_/Cancer Research UK/United Kingdom
- BB/I001794/1/BB_/Biotechnology and Biological Sciences Research Council/United Kingdom
- R01 HL112719/HL/NHLBI NIH HHS/United States
- G0800784/MRC_/Medical Research Council/United Kingdom
LinkOut - more resources
Full Text Sources
Other Literature Sources
Molecular Biology Databases
Research Materials
Miscellaneous