Obstructing toxin pathways by targeted pore blockage
- PMID: 23057504
- PMCID: PMC3681896
- DOI: 10.1021/cr300141q
Obstructing toxin pathways by targeted pore blockage
Figures
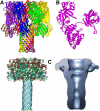
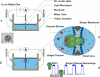
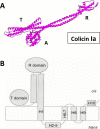
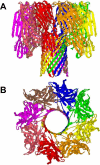
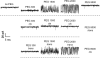
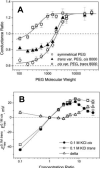
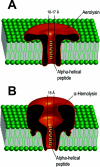
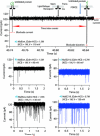
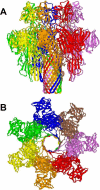
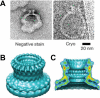
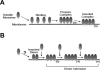
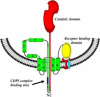
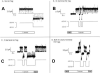
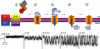
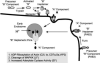
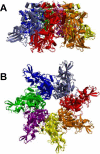
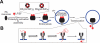
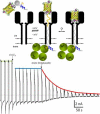
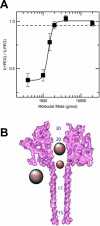
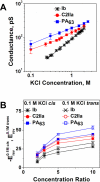
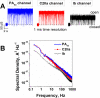
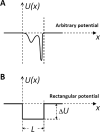
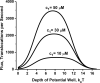
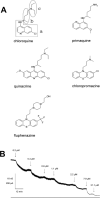
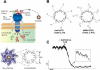
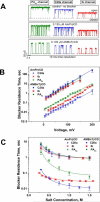
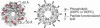
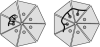
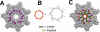
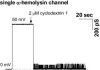
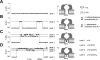
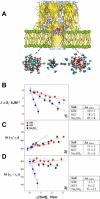
Similar articles
-
Pore-forming toxins.Cell Mol Life Sci. 2002 May;59(5):832-44. doi: 10.1007/s00018-002-8471-1. Cell Mol Life Sci. 2002. PMID: 12088283 Free PMC article. Review.
-
Inhibiting bacterial toxins by channel blockage.Pathog Dis. 2016 Mar;74(2):ftv113. doi: 10.1093/femspd/ftv113. Epub 2015 Dec 9. Pathog Dis. 2016. PMID: 26656888 Free PMC article. Review.
-
Insertion and organization within membranes of the delta-endotoxin pore-forming domain, helix 4-loop-helix 5, and inhibition of its activity by a mutant helix 4 peptide.J Biol Chem. 2000 Aug 4;275(31):23602-7. doi: 10.1074/jbc.M002596200. J Biol Chem. 2000. PMID: 10811807
-
Molecular features of the cytolytic pore-forming bacterial protein toxins.Folia Microbiol (Praha). 2003;48(1):5-16. doi: 10.1007/BF02931271. Folia Microbiol (Praha). 2003. PMID: 12744072 Review.
-
Voltage gating is a fundamental feature of porin and toxin beta-barrel membrane channels.FEBS Lett. 1998 Jul 24;431(3):305-8. doi: 10.1016/s0014-5793(98)00761-3. FEBS Lett. 1998. PMID: 9714531 Review.
Cited by
-
Broad-spectrum and powerful neutralization of bacterial toxins by erythroliposomes with the help of macrophage uptake and degradation.Acta Pharm Sin B. 2022 Nov;12(11):4235-4248. doi: 10.1016/j.apsb.2022.03.015. Epub 2022 Mar 29. Acta Pharm Sin B. 2022. PMID: 36386467 Free PMC article.
-
Multivalent Inhibitors of Channel-Forming Bacterial Toxins.Curr Top Microbiol Immunol. 2017;406:199-227. doi: 10.1007/82_2016_20. Curr Top Microbiol Immunol. 2017. PMID: 27469304 Free PMC article. Review.
-
Design of monodisperse and well-defined polypeptide-based polyvalent inhibitors of anthrax toxin.Angew Chem Int Ed Engl. 2014 Jul 28;53(31):8037-40. doi: 10.1002/anie.201400870. Epub 2014 Apr 6. Angew Chem Int Ed Engl. 2014. PMID: 24706570 Free PMC article.
-
The Transmembrane Domain of a Bicomponent ABC Transporter Exhibits Channel-Forming Activity.ACS Chem Biol. 2016 Sep 16;11(9):2506-18. doi: 10.1021/acschembio.6b00383. Epub 2016 Jul 19. ACS Chem Biol. 2016. PMID: 27379442 Free PMC article.
-
Probing Translocation in Mutants of the Anthrax Channel: Atomically Detailed Simulations with Milestoning.J Phys Chem B. 2018 Nov 15;122(45):10296-10305. doi: 10.1021/acs.jpcb.8b08304. Epub 2018 Nov 5. J Phys Chem B. 2018. PMID: 30338689 Free PMC article.
References
Publication types
MeSH terms
Substances
Grants and funding
LinkOut - more resources
Full Text Sources