Interactions of nanomaterials and biological systems: Implications to personalized nanomedicine
- PMID: 22917779
- PMCID: PMC3517211
- DOI: 10.1016/j.addr.2012.08.005
Interactions of nanomaterials and biological systems: Implications to personalized nanomedicine
Abstract
The application of nanotechnology to personalized medicine provides an unprecedented opportunity to improve the treatment of many diseases. Nanomaterials offer several advantages as therapeutic and diagnostic tools due to design flexibility, small sizes, large surface-to-volume ratio, and ease of surface modification with multivalent ligands to increase avidity for target molecules. Nanomaterials can be engineered to interact with specific biological components, allowing them to benefit from the insights provided by personalized medicine techniques. To tailor these interactions, a comprehensive knowledge of how nanomaterials interact with biological systems is critical. Herein, we discuss how the interactions of nanomaterials with biological systems can guide their design for diagnostic, imaging and drug delivery purposes. A general overview of nanomaterials under investigation is provided with an emphasis on systems that have reached clinical trials. Finally, considerations for the development of personalized nanomedicines are summarized such as the potential toxicity, scientific and technical challenges in fabricating them, and regulatory and ethical issues raised by the utilization of nanomaterials.
Copyright © 2012 Elsevier B.V. All rights reserved.
Figures
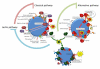
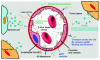
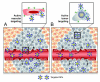
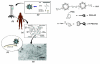
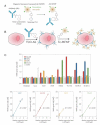
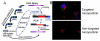
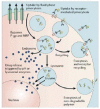
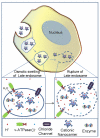
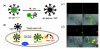
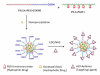
Similar articles
-
Antibody-guided nanomedicines as novel breakthrough therapeutic, diagnostic and theranostic tools.Biomater Sci. 2019 Oct 1;7(10):4000-4016. doi: 10.1039/c9bm00931k. Epub 2019 Jul 29. Biomater Sci. 2019. PMID: 31355391 Review.
-
Challenges in the development of nanoparticle-based imaging agents: Characterization and biology.Wiley Interdiscip Rev Nanomed Nanobiotechnol. 2021 Jan;13(1):e1665. doi: 10.1002/wnan.1665. Epub 2020 Aug 23. Wiley Interdiscip Rev Nanomed Nanobiotechnol. 2021. PMID: 32830448 Review.
-
2D Nanomaterials for Cancer Theranostic Applications.Adv Mater. 2020 Apr;32(13):e1902333. doi: 10.1002/adma.201902333. Epub 2019 Jul 28. Adv Mater. 2020. PMID: 31353752 Review.
-
Theranostic nanomedicine.Acc Chem Res. 2011 Oct 18;44(10):1029-38. doi: 10.1021/ar200019c. Epub 2011 May 5. Acc Chem Res. 2011. PMID: 21545096 Review.
-
Nanomedicines: addressing the scientific and regulatory gap.Ann N Y Acad Sci. 2014 Apr;1313:35-56. doi: 10.1111/nyas.12403. Epub 2014 Mar 27. Ann N Y Acad Sci. 2014. PMID: 24673240
Cited by
-
Preventing diet-induced obesity in mice by adipose tissue transformation and angiogenesis using targeted nanoparticles.Proc Natl Acad Sci U S A. 2016 May 17;113(20):5552-7. doi: 10.1073/pnas.1603840113. Epub 2016 May 2. Proc Natl Acad Sci U S A. 2016. PMID: 27140638 Free PMC article.
-
Cancer nanomedicine: from targeted delivery to combination therapy.Trends Mol Med. 2015 Apr;21(4):223-32. doi: 10.1016/j.molmed.2015.01.001. Epub 2015 Feb 2. Trends Mol Med. 2015. PMID: 25656384 Free PMC article. Review.
-
Poly lactic-co-glycolic acid controlled delivery of disulfiram to target liver cancer stem-like cells.Nanomedicine. 2017 Feb;13(2):641-657. doi: 10.1016/j.nano.2016.08.001. Epub 2016 Aug 10. Nanomedicine. 2017. PMID: 27521693 Free PMC article.
-
Nanomedicine: Principles, Properties, and Regulatory Issues.Front Chem. 2018 Aug 20;6:360. doi: 10.3389/fchem.2018.00360. eCollection 2018. Front Chem. 2018. PMID: 30177965 Free PMC article. Review.
-
Targeted therapy using nanotechnology: focus on cancer.Int J Nanomedicine. 2014 Jan 15;9:467-83. doi: 10.2147/IJN.S36654. eCollection 2014. Int J Nanomedicine. 2014. PMID: 24531078 Free PMC article. Review.
References
-
- Ferrari M. Cancer nanotechnology: opportunities and challenges. Nat. Rev. Cancer. 2005;5:161–171. - PubMed
-
- Jain KK. Role of nanobiotechnology in the development of personalized medicine. Nanomedicine (Lond) 2009;4:249–252. - PubMed
-
- Jain KK. Personalized medicine. Curr. Opin. Mol. Ther. 2002;4:548–558. - PubMed
-
- Vizirianakis IS. Nanomedicine and personalized medicine toward the application of pharmacotyping in clinical practice to improve drug-delivery outcomes. Nanomedicine. 2011;7:11–17. - PubMed
-
- Sakamoto JH, van de Ven AL, Godin B, Blanco E, Serda RE, Grattoni A, Ziemys A, Bouamrani A, Hu T, Ranganathan SI, De Rosa E, Martinez JO, Smid CA, Buchanan RM, Lee SY, Srinivasan S, Landry M, Meyn A, Tasciotti E, Liu X, Decuzzi P, Ferrari M. Enabling individualized therapy through nanotechnology. Pharmacol. Res. 2010;62:57–89. - PMC - PubMed
Publication types
MeSH terms
Substances
Grants and funding
LinkOut - more resources
Full Text Sources
Other Literature Sources