GFAP isoforms in adult mouse brain with a focus on neurogenic astrocytes and reactive astrogliosis in mouse models of Alzheimer disease
- PMID: 22912745
- PMCID: PMC3418292
- DOI: 10.1371/journal.pone.0042823
GFAP isoforms in adult mouse brain with a focus on neurogenic astrocytes and reactive astrogliosis in mouse models of Alzheimer disease
Abstract
Glial fibrillary acidic protein (GFAP) is the main astrocytic intermediate filament (IF). GFAP splice isoforms show differential expression patterns in the human brain. GFAPδ is preferentially expressed by neurogenic astrocytes in the subventricular zone (SVZ), whereas GFAP(+1) is found in a subset of astrocytes throughout the brain. In addition, the expression of these isoforms in human brain material of epilepsy, Alzheimer and glioma patients has been reported. Here, for the first time, we present a comprehensive study of GFAP isoform expression in both wild-type and Alzheimer Disease (AD) mouse models. In cortex, cerebellum, and striatum of wild-type mice, transcripts for Gfap-α, Gfap-β, Gfap-γ, Gfap-δ, Gfap-κ, and a newly identified isoform Gfap-ζ, were detected. Their relative expression levels were similar in all regions studied. GFAPα showed a widespread expression whilst GFAPδ distribution was prominent in the SVZ, rostral migratory stream (RMS), neurogenic astrocytes of the subgranular zone (SGZ), and subpial astrocytes. In contrast to the human SVZ, we could not establish an unambiguous GFAPδ localization in proliferating cells of the mouse SVZ. In APPswePS1dE9 and 3xTgAD mice, plaque-associated reactive astrocytes had increased transcript levels of all detectable GFAP isoforms and low levels of a new GFAP isoform, Gfap-ΔEx7. Reactive astrocytes in AD mice showed enhanced GFAPα and GFAPδ immunolabeling, less frequently increased vimentin and nestin, but no GFAPκ or GFAP(+1) staining. In conclusion, GFAPδ protein is present in SVZ, RMS, and neurogenic astrocytes of the SGZ, but also outside neurogenic niches. Furthermore, differential GFAP isoform expression is not linked with aging or reactive gliosis. This evidence points to the conclusion that differential regulation of GFAP isoforms is not involved in the reorganization of the IF network in reactive gliosis or in neurogenesis in the mouse brain.
Conflict of interest statement
Figures
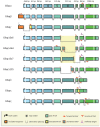
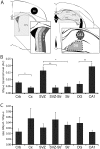
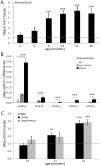
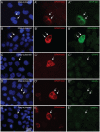
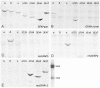
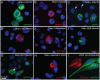
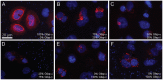
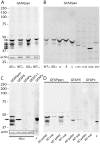
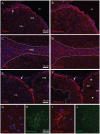
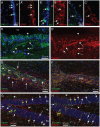
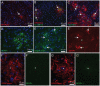
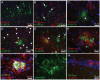
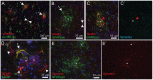
Similar articles
-
Glial fibrillary acidic protein isoform expression in plaque related astrogliosis in Alzheimer's disease.Neurobiol Aging. 2014 Mar;35(3):492-510. doi: 10.1016/j.neurobiolaging.2013.09.035. Epub 2013 Oct 23. Neurobiol Aging. 2014. PMID: 24269023
-
Alternative mRNA splicing from the glial fibrillary acidic protein (GFAP) gene generates isoforms with distinct subcellular mRNA localization patterns in astrocytes.PLoS One. 2013 Aug 26;8(8):e72110. doi: 10.1371/journal.pone.0072110. eCollection 2013. PLoS One. 2013. PMID: 23991052 Free PMC article.
-
Adult human subventricular, subgranular, and subpial zones contain astrocytes with a specialized intermediate filament cytoskeleton.Glia. 2005 Dec;52(4):289-300. doi: 10.1002/glia.20243. Glia. 2005. PMID: 16001427
-
GFAP Alternative Splicing and the Relevance for Disease - A Focus on Diffuse Gliomas.ASN Neuro. 2022 Jan-Dec;14:17590914221102065. doi: 10.1177/17590914221102065. ASN Neuro. 2022. PMID: 35673702 Free PMC article. Review.
-
GFAP in health and disease.Prog Neurobiol. 2011 Mar;93(3):421-43. doi: 10.1016/j.pneurobio.2011.01.005. Epub 2011 Jan 8. Prog Neurobiol. 2011. PMID: 21219963 Review.
Cited by
-
Pathology associated with AAV mediated expression of beta amyloid or C100 in adult mouse hippocampus and cerebellum.PLoS One. 2013;8(3):e59166. doi: 10.1371/journal.pone.0059166. Epub 2013 Mar 13. PLoS One. 2013. PMID: 23516609 Free PMC article.
-
Age-Related Cognitive and Motor Decline in a Mouse Model of CDKL5 Deficiency Disorder is Associated with Increased Neuronal Senescence and Death.Aging Dis. 2021 Jun 1;12(3):764-785. doi: 10.14336/AD.2020.0827. eCollection 2021 Jun. Aging Dis. 2021. PMID: 34094641 Free PMC article.
-
Endogenous cerebellar neurogenesis in adult mice with progressive ataxia.Ann Clin Transl Neurol. 2014 Dec;1(12):968-81. doi: 10.1002/acn3.137. Epub 2014 Nov 4. Ann Clin Transl Neurol. 2014. PMID: 25574472 Free PMC article.
-
Exercise suppresses neuroinflammation for alleviating Alzheimer's disease.J Neuroinflammation. 2023 Mar 19;20(1):76. doi: 10.1186/s12974-023-02753-6. J Neuroinflammation. 2023. PMID: 36935511 Free PMC article. Review.
-
Astrocytes in human central nervous system diseases: a frontier for new therapies.Signal Transduct Target Ther. 2023 Oct 13;8(1):396. doi: 10.1038/s41392-023-01628-9. Signal Transduct Target Ther. 2023. PMID: 37828019 Free PMC article. Review.
References
-
- Seth P, Koul N (2008) Astrocyte, the star avatar: redefined. J Biosci 33: 405–421. - PubMed
-
- Oberheim NA, Goldman SA, Nedergaard M (2012) Heterogeneity of astrocyte form and function. In: Walker JM, editors. Astrocytes. Methods and Protocols. Humana Press. 23–45.
-
- Doetsch F, Caille I, Lim DA, Garcia-Verdugo JM, Varez-Buylla A (1999) Subventricular zone astrocytes are neural stem cells in the adult mammalian brain. Cell 97: 703–716. - PubMed
Publication types
MeSH terms
Substances
Grants and funding
LinkOut - more resources
Full Text Sources
Other Literature Sources
Medical
Molecular Biology Databases
Miscellaneous