Mechanistic characterization of the 5'-triphosphate-dependent activation of PKR: lack of 5'-end nucleobase specificity, evidence for a distinct triphosphate binding site, and a critical role for the dsRBD
- PMID: 22912486
- PMCID: PMC3446709
- DOI: 10.1261/rna.034520.112
Mechanistic characterization of the 5'-triphosphate-dependent activation of PKR: lack of 5'-end nucleobase specificity, evidence for a distinct triphosphate binding site, and a critical role for the dsRBD
Abstract
The protein kinase PKR is activated by RNA to phosphorylate eIF-2α, inhibiting translation initiation. Long dsRNA activates PKR via interactions with the dsRNA-binding domain (dsRBD). Weakly structured RNA also activates PKR and does so in a 5'-triphosphate (ppp)-dependent fashion, however relatively little is known about this pathway. We used a mutant T7 RNA polymerase to incorporate all four triphosphate-containing nucleotides into the first position of a largely single-stranded RNA and found absence of selectivity, in that all four transcripts activate PKR. Recognition of 5'-triphosphate, but not the nucleobase at the 5'-most position, makes this RNA-mediated innate immune response sensitive to a broad array of viruses. PKR was neither activated in the presence of γ-GTP nor recognized NTPs other than ATP in activation competition and ITC binding assays. This indicates that the binding site for ATP is selective, which contrasts with the site for the 5' end of ppp-ssRNA. Activation experiments reveal that short dsRNAs compete with 5'-triphosphate RNAs and heparin for activation, and likewise gel-shift assays reveal that activating 5'-triphosphate RNAs and heparin compete with short dsRNAs for binding to PKR's dsRBD. The dsRBD thus plays a critical role in the activation of PKR by ppp-ssRNA and even heparin. At the same time, cross-linking experiments indicate that ppp-ssRNA interacts with PKR outside of the dsRBD as well. Overall, 5'-triphosphate-containing, weakly structured RNAs activate PKR via interactions with both the dsRBD and a distinct triphosphate binding site that lacks 5'-nucleobase specificity, allowing the innate immune response to provide broad-spectrum protection from pathogens.
Figures
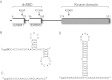
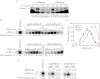
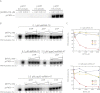
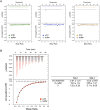
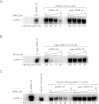
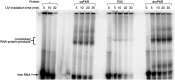
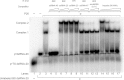
Similar articles
-
Discriminating Self and Non-Self by RNA: Roles for RNA Structure, Misfolding, and Modification in Regulating the Innate Immune Sensor PKR.Acc Chem Res. 2016 Jun 21;49(6):1242-9. doi: 10.1021/acs.accounts.6b00151. Epub 2016 Jun 8. Acc Chem Res. 2016. PMID: 27269119 Free PMC article. Review.
-
Nucleoside modifications modulate activation of the protein kinase PKR in an RNA structure-specific manner.RNA. 2008 Jun;14(6):1201-13. doi: 10.1261/rna.1007408. Epub 2008 Apr 21. RNA. 2008. PMID: 18426922 Free PMC article.
-
Identification of binding sites for both dsRBMs of PKR on kinase-activating and kinase-inhibiting RNA ligands.Biochemistry. 2002 Apr 9;41(14):4511-20. doi: 10.1021/bi0120594. Biochemistry. 2002. PMID: 11926812
-
Uncoupling of RNA binding and PKR kinase activation by viral inhibitor RNAs.J Mol Biol. 2006 May 19;358(5):1270-85. doi: 10.1016/j.jmb.2006.03.003. Epub 2006 Mar 20. J Mol Biol. 2006. PMID: 16580685
-
Viral proteins targeting host protein kinase R to evade an innate immune response: a mini review.Biotechnol Genet Eng Rev. 2018 Apr;34(1):33-59. doi: 10.1080/02648725.2018.1467151. Epub 2018 May 2. Biotechnol Genet Eng Rev. 2018. PMID: 29716441 Review.
Cited by
-
Cytidine acetylation yields a hypoinflammatory synthetic messenger RNA.Cell Chem Biol. 2022 Feb 17;29(2):312-320.e7. doi: 10.1016/j.chembiol.2021.07.003. Epub 2021 Aug 25. Cell Chem Biol. 2022. PMID: 35180432 Free PMC article.
-
Native tertiary structure and nucleoside modifications suppress tRNA's intrinsic ability to activate the innate immune sensor PKR.PLoS One. 2013;8(3):e57905. doi: 10.1371/journal.pone.0057905. Epub 2013 Mar 4. PLoS One. 2013. PMID: 23483938 Free PMC article.
-
Discriminating Self and Non-Self by RNA: Roles for RNA Structure, Misfolding, and Modification in Regulating the Innate Immune Sensor PKR.Acc Chem Res. 2016 Jun 21;49(6):1242-9. doi: 10.1021/acs.accounts.6b00151. Epub 2016 Jun 8. Acc Chem Res. 2016. PMID: 27269119 Free PMC article. Review.
-
The search for a PKR code-differential regulation of protein kinase R activity by diverse RNA and protein regulators.RNA. 2019 May;25(5):539-556. doi: 10.1261/rna.070169.118. Epub 2019 Feb 15. RNA. 2019. PMID: 30770398 Free PMC article. Review.
-
Ribosome changes reprogram translation for chemosurvival in G0 leukemic cells.Sci Adv. 2022 Oct 28;8(43):eabo1304. doi: 10.1126/sciadv.abo1304. Epub 2022 Oct 28. Sci Adv. 2022. PMID: 36306353 Free PMC article.
References
-
- Bevilacqua PC, Cech TR 1996. Minor-groove recognition of double-stranded RNA by the double-stranded RNA-binding domain from the RNA-activated protein kinase PKR. Biochemistry 35: 9983–9994 - PubMed
Publication types
MeSH terms
Substances
Grants and funding
LinkOut - more resources
Full Text Sources
Other Literature Sources