End-on microtubule-dynein interactions and pulling-based positioning of microtubule organizing centers
- PMID: 22895049
- PMCID: PMC3495818
- DOI: 10.4161/cc.21753
End-on microtubule-dynein interactions and pulling-based positioning of microtubule organizing centers
Abstract
During important cellular processes such as centrosome and spindle positioning, dynein at the cortex interacts with dynamic microtubules in an apparent "end-on" fashion. It is well-established that dynein can generate forces by moving laterally along the microtubule lattice, but much less is known about dynein's interaction with dynamic microtubule ends. In this paper, we review recent in vitro experiments that show that dynein, attached to an artificial cortex, is able to capture microtubule ends, regulate microtubule dynamics and mediate the generation of pulling forces on shrinking microtubules. We further review existing ideas on the involvement of dynein-mediated cortical pulling forces in the positioning of microtubule organizing centers such as centrosomes. Recent in vitro experiments have demonstrated that cortical pulling forces in combination with pushing forces can lead to reliable centering of microtubule asters in quasi two-dimensional microfabricated chambers. In these experiments, pushing leads to slipping of microtubule ends along the chamber boundaries, resulting in an anisotropic distribution of cortical microtubule contacts that favors centering, once pulling force generators become engaged. This effect is predicted to be strongly geometry-dependent, and we therefore finally discuss ongoing efforts to repeat these experiments in three-dimensional, spherical and deformable geometries.
Figures
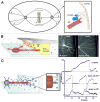
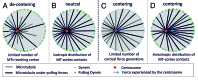
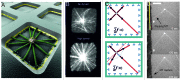
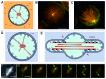
Similar articles
-
Cortical dynein controls microtubule dynamics to generate pulling forces that position microtubule asters.Cell. 2012 Feb 3;148(3):502-14. doi: 10.1016/j.cell.2012.01.007. Cell. 2012. PMID: 22304918 Free PMC article.
-
Visualization of dynein-dependent microtubule gliding at the cell cortex: implications for spindle positioning.J Cell Biol. 2011 Aug 8;194(3):377-86. doi: 10.1083/jcb.201103128. J Cell Biol. 2011. PMID: 21825072 Free PMC article.
-
Effects of dynein on microtubule mechanics and centrosome positioning.Mol Biol Cell. 2011 Dec;22(24):4834-41. doi: 10.1091/mbc.E11-07-0611. Epub 2011 Oct 19. Mol Biol Cell. 2011. PMID: 22013075 Free PMC article.
-
Physical Limits on the Precision of Mitotic Spindle Positioning by Microtubule Pushing forces: Mechanics of mitotic spindle positioning.Bioessays. 2017 Nov;39(11):10.1002/bies.201700122. doi: 10.1002/bies.201700122. Epub 2017 Sep 28. Bioessays. 2017. PMID: 28960439 Free PMC article. Review.
-
The cortical force-generating machinery: how cortical spindle-pulling forces are generated.Curr Opin Cell Biol. 2019 Oct;60:1-8. doi: 10.1016/j.ceb.2019.03.001. Epub 2019 Apr 5. Curr Opin Cell Biol. 2019. PMID: 30954860 Review.
Cited by
-
Positioning centrioles and centrosomes.J Cell Biol. 2024 Apr 1;223(4):e202311140. doi: 10.1083/jcb.202311140. Epub 2024 Mar 21. J Cell Biol. 2024. PMID: 38512059 Free PMC article. Review.
-
The GTP-tubulin cap is not the determinant of microtubule end stability in cells.Mol Biol Cell. 2024 Oct 1;35(10):br19. doi: 10.1091/mbc.E24-07-0307. Epub 2024 Sep 11. Mol Biol Cell. 2024. PMID: 39259768 Free PMC article.
-
The novel actin/focal adhesion-associated protein MISP is involved in mitotic spindle positioning in human cells.Cell Cycle. 2013 May 1;12(9):1457-71. doi: 10.4161/cc.24602. Epub 2013 Apr 10. Cell Cycle. 2013. PMID: 23574715 Free PMC article.
-
The Spindle: Integrating Architecture and Mechanics across Scales.Trends Cell Biol. 2018 Nov;28(11):896-910. doi: 10.1016/j.tcb.2018.07.003. Epub 2018 Aug 6. Trends Cell Biol. 2018. PMID: 30093097 Free PMC article. Review.
-
Motor-driven marginal band coiling promotes cell shape change during platelet activation.J Cell Biol. 2014 Jan 20;204(2):177-85. doi: 10.1083/jcb.201306085. Epub 2014 Jan 13. J Cell Biol. 2014. PMID: 24421335 Free PMC article.
References
Publication types
MeSH terms
Substances
LinkOut - more resources
Full Text Sources
Molecular Biology Databases