Mesenchymal stem cell-mediated reversal of bronchopulmonary dysplasia and associated pulmonary hypertension
- PMID: 22837858
- PMCID: PMC3401871
- DOI: 10.4103/2045-8932.97603
Mesenchymal stem cell-mediated reversal of bronchopulmonary dysplasia and associated pulmonary hypertension
Abstract
Clinical trials have failed to demonstrate an effective preventative or therapeutic strategy for bronchopulmonary dysplasia (BPD), a multifactorial chronic lung disease in preterm infants frequently complicated by pulmonary hypertension (PH). Mesenchymal stem cells (MSCs) and their secreted components have been shown to prevent BPD and pulmonary fibrosis in rodent models. We hypothesized that treatment with conditioned media (CM) from cultured mouse bone marrow-derived MSCs could reverse hyperoxia-induced BPD and PH. Newborn mice were exposed to hyperoxia (FiO(2)=0.75) for two weeks, were then treated with one intravenous dose of CM from either MSCs or primary mouse lung fibroblasts (MLFs), and placed in room air for two to four weeks. Histological analysis of lungs harvested at four weeks of age was performed to determine the degree of alveolar injury, blood vessel number, and vascular remodeling. At age six weeks, pulmonary artery pressure (PA acceleration time) and right ventricular hypertrophy (RVH; RV wall thickness) were assessed by echocardiography, and pulmonary function tests were conducted. When compared to MLF-CM, a single dose of MSC-CM-treatment (1) reversed the hyperoxia-induced parenchymal fibrosis and peripheral PA devascularization (pruning), (2) partially reversed alveolar injury, (3) normalized lung function (airway resistance, dynamic lung compliance), (4) fully reversed the moderate PH and RVH, and (5) attenuated peripheral PA muscularization associated with hyperoxia-induced BPD. Reversal of key features of hyperoxia-induced BPD and its long-term adverse effects on lung function can be achieved by a single intravenous dose of MSC-CM, thereby pointing toward a new therapeutic intervention for chronic lung diseases.
Keywords: airway hyperresponsiveness; chronic lung disease of infancy; hyperoxia; lung injury; lung vascular pruning.
Conflict of interest statement
Figures
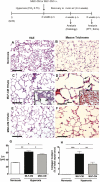
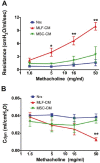
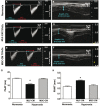
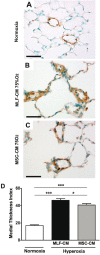
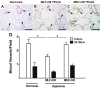
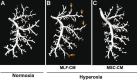
Similar articles
-
Early gestational mesenchymal stem cell secretome attenuates experimental bronchopulmonary dysplasia in part via exosome-associated factor TSG-6.Stem Cell Res Ther. 2018 Jun 26;9(1):173. doi: 10.1186/s13287-018-0903-4. Stem Cell Res Ther. 2018. PMID: 29941022 Free PMC article.
-
Nintedanib preserves lung growth and prevents pulmonary hypertension in a hyperoxia-induced lung injury model.Pediatr Res. 2024 Oct 11. doi: 10.1038/s41390-024-03562-0. Online ahead of print. Pediatr Res. 2024. PMID: 39394424
-
Comparative Effects of Bone Marrow-derived Versus Umbilical Cord Tissue Mesenchymal Stem Cells in an Experimental Model of Bronchopulmonary Dysplasia.Stem Cells Transl Med. 2022 Mar 17;11(2):189-199. doi: 10.1093/stcltm/szab011. Stem Cells Transl Med. 2022. PMID: 35298658 Free PMC article.
-
Therapeutic potential of mesenchymal stem cells for pulmonary complications associated with preterm birth.Int J Biochem Cell Biol. 2016 May;74:18-32. doi: 10.1016/j.biocel.2016.02.023. Epub 2016 Feb 27. Int J Biochem Cell Biol. 2016. PMID: 26928452 Review.
-
Mesenchymal stromal/stem cells and bronchopulmonary dysplasia.Front Cell Dev Biol. 2023 Oct 30;11:1247339. doi: 10.3389/fcell.2023.1247339. eCollection 2023. Front Cell Dev Biol. 2023. PMID: 37965579 Free PMC article. Review.
Cited by
-
Mesenchymal Stromal Cell-Derived Extracellular Vesicles Restore Thymic Architecture and T Cell Function Disrupted by Neonatal Hyperoxia.Front Immunol. 2021 Apr 15;12:640595. doi: 10.3389/fimmu.2021.640595. eCollection 2021. Front Immunol. 2021. PMID: 33936055 Free PMC article.
-
Therapeutic potential of mesenchymal stem/stromal cell-derived secretome and vesicles for lung injury and disease.Expert Opin Biol Ther. 2020 Feb;20(2):125-140. doi: 10.1080/14712598.2020.1689954. Epub 2019 Nov 18. Expert Opin Biol Ther. 2020. PMID: 31701782 Free PMC article. Review.
-
Decorin Secreted by Human Umbilical Cord Blood-Derived Mesenchymal Stem Cells Induces Macrophage Polarization via CD44 to Repair Hyperoxic Lung Injury.Int J Mol Sci. 2019 Sep 27;20(19):4815. doi: 10.3390/ijms20194815. Int J Mol Sci. 2019. PMID: 31569732 Free PMC article.
-
Adrenomedullin Is Necessary to Resolve Hyperoxia-Induced Experimental Bronchopulmonary Dysplasia and Pulmonary Hypertension in Mice.Am J Pathol. 2020 Mar;190(3):711-722. doi: 10.1016/j.ajpath.2019.11.011. Epub 2020 Feb 21. Am J Pathol. 2020. PMID: 32093901 Free PMC article.
-
MSC microvesicles for the treatment of lung disease: a new paradigm for cell-free therapy.Antioxid Redox Signal. 2014 Nov 1;21(13):1905-15. doi: 10.1089/ars.2013.5784. Epub 2014 Feb 24. Antioxid Redox Signal. 2014. PMID: 24382303 Free PMC article. Review.
References
-
- Jobe AH, Bancalari E. Bronchopulmonary dysplasia. Am J Respir Crit Care Med. 2001;163:1723–9. - PubMed
-
- Stenmark KR, Abman SH. Lung vascular development: implications for the pathogenesis of bronchopulmonary dysplasia. Annu Rev Physiol. 2005;67:623–61. - PubMed
-
- Baraldi E, Filippone M. Chronic lung disease after premature birth. N Engl J Med. 2007;357:1946–55. - PubMed
-
- Kim DH, Kim HS, Choi CW, Kim EK, Kim BI, Choi JH. Risk factors for pulmonary artery hypertension in preterm infants with moderate or severe bronchopulmonary dysplasia. Neonatology. 2012;101:40–6. - PubMed
Grants and funding
LinkOut - more resources
Full Text Sources
Other Literature Sources