Crystal structure of the conserved domain of the DC lysosomal associated membrane protein: implications for the lysosomal glycocalyx
- PMID: 22809326
- PMCID: PMC3409847
- DOI: 10.1186/1741-7007-10-62
Crystal structure of the conserved domain of the DC lysosomal associated membrane protein: implications for the lysosomal glycocalyx
Abstract
Background: The family of lysosome-associated membrane proteins (LAMP) comprises the multifunctional, ubiquitous LAMP-1 and LAMP-2, and the cell type-specific proteins DC-LAMP (LAMP-3), BAD-LAMP (UNC-46, C20orf103) and macrosialin (CD68). LAMPs have been implicated in a multitude of cellular processes, including phagocytosis, autophagy, lipid transport and aging. LAMP-2 isoform A acts as a receptor in chaperone-mediated autophagy. LAMP-2 deficiency causes the fatal Danon disease. The abundant proteins LAMP-1 and LAMP-2 are major constituents of the glycoconjugate coat present on the inside of the lysosomal membrane, the 'lysosomal glycocalyx'. The LAMP family is characterized by a conserved domain of 150 to 200 amino acids with two disulfide bonds.
Results: The crystal structure of the conserved domain of human DC-LAMP was solved. It is the first high-resolution structure of a heavily glycosylated lysosomal membrane protein. The structure represents a novel β-prism fold formed by two β-sheets bent by β-bulges and connected by a disulfide bond. Flexible loops and a hydrophobic pocket represent possible sites of molecular interaction. Computational models of the glycosylated luminal regions of LAMP-1 and LAMP-2 indicate that the proteins adopt a compact conformation in close proximity to the lysosomal membrane. The models correspond to the thickness of the lysosomal glycoprotein coat of only 5 to 12 nm, according to electron microscopy.
Conclusion: The conserved luminal domain of lysosome-associated membrane proteins forms a previously unknown β-prism fold. Insights into the structure of the lysosomal glycoprotein coat were obtained by computational models of the LAMP-1 and LAMP-2 luminal regions.
Figures
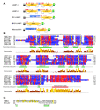

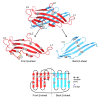
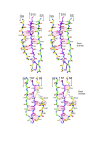
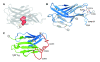
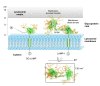
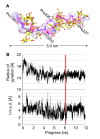
Similar articles
-
Lysosome-associated membrane proteins-1 and -2 (LAMP-1 and LAMP-2) assemble via distinct modes.Biochem Biophys Res Commun. 2016 Oct 21;479(3):489-495. doi: 10.1016/j.bbrc.2016.09.093. Epub 2016 Sep 20. Biochem Biophys Res Commun. 2016. PMID: 27663661
-
At the acidic edge: emerging functions for lysosomal membrane proteins.Trends Cell Biol. 2003 Mar;13(3):137-45. doi: 10.1016/s0962-8924(03)00005-9. Trends Cell Biol. 2003. PMID: 12628346 Review.
-
Lysosome membrane lipid microdomains: novel regulators of chaperone-mediated autophagy.EMBO J. 2006 Sep 6;25(17):3921-33. doi: 10.1038/sj.emboj.7601283. Epub 2006 Aug 17. EMBO J. 2006. PMID: 16917501 Free PMC article.
-
The targeting of cystinosin to the lysosomal membrane requires a tyrosine-based signal and a novel sorting motif.J Biol Chem. 2001 Apr 20;276(16):13314-21. doi: 10.1074/jbc.M010562200. Epub 2001 Jan 9. J Biol Chem. 2001. PMID: 11150305
-
Lysosomal membrane proteins.Eur J Paediatr Neurol. 2001;5 Suppl A:11-9. doi: 10.1053/ejpn.2000.0428. Eur J Paediatr Neurol. 2001. PMID: 11588980 Review.
Cited by
-
TSTA3 facilitates esophageal squamous cell carcinoma progression through regulating fucosylation of LAMP2 and ERBB2.Theranostics. 2020 Sep 14;10(24):11339-11358. doi: 10.7150/thno.48225. eCollection 2020. Theranostics. 2020. PMID: 33042286 Free PMC article.
-
Lysosome associated membrane proteins maintain pancreatic acinar cell homeostasis: LAMP-2 deficient mice develop pancreatitis.Cell Mol Gastroenterol Hepatol. 2015 Nov 1;1(6):678-694. doi: 10.1016/j.jcmgh.2015.07.006. Cell Mol Gastroenterol Hepatol. 2015. PMID: 26693174 Free PMC article.
-
Lysosomes in Cancer-At the Crossroad of Good and Evil.Cells. 2024 Mar 5;13(5):459. doi: 10.3390/cells13050459. Cells. 2024. PMID: 38474423 Free PMC article. Review.
-
Hydroxypropyl-beta and -gamma cyclodextrins rescue cholesterol accumulation in Niemann-Pick C1 mutant cell via lysosome-associated membrane protein 1.Cell Death Dis. 2018 Oct 3;9(10):1019. doi: 10.1038/s41419-018-1056-1. Cell Death Dis. 2018. PMID: 30282967 Free PMC article.
-
Determining if T cell antigens are naturally processed and presented on HLA class I molecules.BMC Immunol. 2022 Feb 11;23(1):5. doi: 10.1186/s12865-022-00478-4. BMC Immunol. 2022. PMID: 35148673 Free PMC article.
References
Publication types
MeSH terms
Substances
Associated data
- Actions
LinkOut - more resources
Full Text Sources
Other Literature Sources
Molecular Biology Databases
Research Materials
Miscellaneous