Mechanisms of tumor vascular priming by a nanoparticulate doxorubicin formulation
- PMID: 22798260
- PMCID: PMC3631713
- DOI: 10.1007/s11095-012-0823-4
Mechanisms of tumor vascular priming by a nanoparticulate doxorubicin formulation
Abstract
Purpose: Tumor vascular normalization by antiangiogenic agents may increase tumor perfusion but reestablish vascular barrier properties in CNS tumors. Vascular priming via nanoparticulate carriers represents a mechanistically distinct alternative. This study investigated mechanisms by which sterically-stabilized liposomal doxorubicin (SSL-DXR) modulates tumor vascular properties.
Methods: Functional vascular responses to SSL-DXR were investigated in orthotopic rat brain tumors using deposition of fluorescent permeability probes and dynamic contrast-enhanced magnetic resonance imaging. Microvessel density and tumor burden were quantified by immunohistochemistry (CD-31) and quantitative RT-PCR (VE-cadherin).
Results: Administration of SSL-DXR (5.7 mg/kg iv) initially (3-4 days post-treatment) decreased tumor vascular permeability, k(trans) (vascular exchange constant), vascular endothelial cell content, microvessel density, and deposition of nanoparticulates. Tumor vasculature became less chaotic. Permeability and perfusion returned to control values 6-7 days post-treatment, but intratumor SSL-DXR depot continued to effect tumor vascular endothelial compartment 7-10 days post-treatment, mediating enhanced permeability.
Conclusions: SSL-DXR ultimately increased tumor vascular permeability, but initially normalized tumor vasculature and decreased tumor perfusion, permeability, and nanoparticulate deposition. These temporal changes in vascular integrity resulting from a single SSL-DXR dose have important implications for the design of combination therapies incorporating nanoparticle-based agents for tumor vascular priming.
Figures
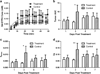
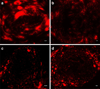
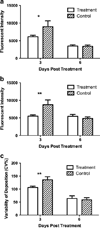
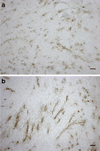
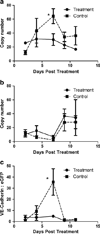
Similar articles
-
Tumor-Priming Smoothened Inhibitor Enhances Deposition and Efficacy of Cytotoxic Nanoparticles in a Pancreatic Cancer Model.Mol Cancer Ther. 2016 Jan;15(1):84-93. doi: 10.1158/1535-7163.MCT-15-0602. Epub 2015 Oct 29. Mol Cancer Ther. 2016. PMID: 26516158 Free PMC article.
-
Effect of repetitive administration of Doxorubicin-containing liposomes on plasma pharmacokinetics and drug biodistribution in a rat brain tumor model.Clin Cancer Res. 2005 Dec 15;11(24 Pt 1):8856-65. doi: 10.1158/1078-0432.CCR-05-1365. Clin Cancer Res. 2005. PMID: 16361575 Free PMC article.
-
Antivasculature effects of doxorubicin-containing liposomes in an intracranial rat brain tumor model.Cancer Res. 2002 May 1;62(9):2561-6. Cancer Res. 2002. PMID: 11980650
-
Antivascular and antitumor activities of liposome-associated drugs.Anticancer Res. 2004 Mar-Apr;24(2A):397-404. Anticancer Res. 2004. PMID: 15152936 Review.
-
A review of micro- and macrovascular analyses in the assessment of tumor-associated vasculature as visualized by MR.Neuroimage. 2007;37 Suppl 1(Suppl 1):S116-9. doi: 10.1016/j.neuroimage.2007.03.067. Epub 2007 Apr 25. Neuroimage. 2007. PMID: 17512217 Free PMC article. Review.
Cited by
-
Cerebral hypoperfusion-assisted intra-arterial deposition of liposomes in normal and glioma-bearing rats.Neurosurgery. 2015 Jan;76(1):92-100. doi: 10.1227/NEU.0000000000000552. Neurosurgery. 2015. PMID: 25525695 Free PMC article.
-
Improving the distribution of Doxil® in the tumor matrix by depletion of tumor hyaluronan.J Control Release. 2014 Oct 10;191:105-14. doi: 10.1016/j.jconrel.2014.05.019. Epub 2014 May 20. J Control Release. 2014. PMID: 24852095 Free PMC article.
-
Induced Vascular Normalization-Can One Force Tumors to Surrender to a Better Microenvironment?Pharmaceutics. 2023 Jul 26;15(8):2022. doi: 10.3390/pharmaceutics15082022. Pharmaceutics. 2023. PMID: 37631236 Free PMC article. Review.
-
Liposome size and charge optimization for intraarterial delivery to gliomas.Drug Deliv Transl Res. 2016 Jun;6(3):225-33. doi: 10.1007/s13346-016-0294-y. Drug Deliv Transl Res. 2016. PMID: 27091339 Free PMC article.
-
A robust and quantitative method for tracking liposome contents after intravenous administration.J Control Release. 2014 Feb 28;176:86-93. doi: 10.1016/j.jconrel.2013.12.014. Epub 2013 Dec 22. J Control Release. 2014. PMID: 24368300 Free PMC article.
References
-
- Jemal A, Siegel R, Ward E, Hao Y, Xu J, Thun MJ. Cancer statistics, 2009. CA Cancer J Clin. 2009;59:225–249. - PubMed
-
- Ries LAG, Kosary CL, Hankey BF, Miller BA, Clegg L, Edwards BK. Surveillance epidemiology end result (SEER) cancer statistics review 1973–1996. Bethesda: National Cancer Institute. 1999
-
- Packer RJ. Brain tumors in children. Arch Neurol. 1999;56:421–425. - PubMed
-
- Fathallah-Shaykh H. New molecular strategies to cure brain tumors. Arch Neurol. 1999;56:449–453. - PubMed
Publication types
MeSH terms
Substances
Grants and funding
LinkOut - more resources
Full Text Sources
Medical