Vascularized bone tissue engineering: approaches for potential improvement
- PMID: 22765012
- PMCID: PMC3458624
- DOI: 10.1089/ten.TEB.2012.0012
Vascularized bone tissue engineering: approaches for potential improvement
Abstract
Significant advances have been made in bone tissue engineering (TE) in the past decade. However, classical bone TE strategies have been hampered mainly due to the lack of vascularization within the engineered bone constructs, resulting in poor implant survival and integration. In an effort toward clinical success of engineered constructs, new TE concepts have arisen to develop bone substitutes that potentially mimic native bone tissue structure and function. Large tissue replacements have failed in the past due to the slow penetration of the host vasculature, leading to necrosis at the central region of the engineered tissues. For this reason, multiple microscale strategies have been developed to induce and incorporate vascular networks within engineered bone constructs before implantation in order to achieve successful integration with the host tissue. Previous attempts to engineer vascularized bone tissue only focused on the effect of a single component among the three main components of TE (scaffold, cells, or signaling cues) and have only achieved limited success. However, with efforts to improve the engineered bone tissue substitutes, bone TE approaches have become more complex by combining multiple strategies simultaneously. The driving force behind combining various TE strategies is to produce bone replacements that more closely recapitulate human physiology. Here, we review and discuss the limitations of current bone TE approaches and possible strategies to improve vascularization in bone tissue substitutes.
Figures
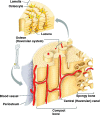
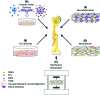
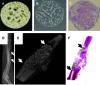
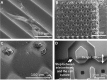
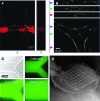
Similar articles
-
Key components of engineering vascularized 3-dimensional bioprinted bone constructs.Transl Res. 2020 Feb;216:57-76. doi: 10.1016/j.trsl.2019.08.010. Epub 2019 Sep 5. Transl Res. 2020. PMID: 31526771 Review.
-
Engineering Pre-vascularized Scaffolds for Bone Regeneration.Adv Exp Med Biol. 2015;881:79-94. doi: 10.1007/978-3-319-22345-2_5. Adv Exp Med Biol. 2015. PMID: 26545745 Review.
-
Vascularization in bone tissue engineering constructs.Ann Biomed Eng. 2015 Mar;43(3):718-29. doi: 10.1007/s10439-015-1253-3. Epub 2015 Jan 24. Ann Biomed Eng. 2015. PMID: 25616591 Free PMC article. Review.
-
Vascularization in bone tissue engineering: physiology, current strategies, major hurdles and future challenges.Macromol Biosci. 2010 Jan 11;10(1):12-27. doi: 10.1002/mabi.200900107. Macromol Biosci. 2010. PMID: 19688722 Review.
-
Review of vascularised bone tissue-engineering strategies with a focus on co-culture systems.J Tissue Eng Regen Med. 2015 Feb;9(2):85-105. doi: 10.1002/term.1617. Epub 2012 Nov 19. J Tissue Eng Regen Med. 2015. PMID: 23166000 Review.
Cited by
-
Vascularization strategies in tissue engineering approaches for soft tissue repair.J Tissue Eng Regen Med. 2021 Sep;15(9):747-762. doi: 10.1002/term.3225. Epub 2021 May 31. J Tissue Eng Regen Med. 2021. PMID: 34058083 Free PMC article. Review.
-
In vivo evaluation of osseointegration ability of sintered bionic trabecular porous titanium alloy as artificial hip prosthesis.Front Bioeng Biotechnol. 2022 Sep 14;10:928216. doi: 10.3389/fbioe.2022.928216. eCollection 2022. Front Bioeng Biotechnol. 2022. PMID: 36185453 Free PMC article.
-
Multifunctionalized carbon-fiber-reinforced polyetheretherketone implant for rapid osseointegration under infected environment.Bioact Mater. 2022 Dec 24;24:236-250. doi: 10.1016/j.bioactmat.2022.12.016. eCollection 2023 Jun. Bioact Mater. 2022. PMID: 36606257 Free PMC article.
-
Stem cell-derived endochondral cartilage stimulates bone healing by tissue transformation.J Bone Miner Res. 2014;29(5):1269-82. doi: 10.1002/jbmr.2148. J Bone Miner Res. 2014. PMID: 24259230 Free PMC article.
-
Phage nanofibers induce vascularized osteogenesis in 3D printed bone scaffolds.Adv Mater. 2014 Aug 6;26(29):4961-4966. doi: 10.1002/adma.201400154. Epub 2014 Apr 7. Adv Mater. 2014. PMID: 24711251 Free PMC article.
References
-
- Salgado A.J. Coutinho O.P. Reis R.L. Bone tissue engineering: state of the art and future trends. Macromol Biosci. 2004;4:743. - PubMed
-
- Goldstein S.A. Tissue engineering: functional assessment and clinical outcome. Ann N Y Acad Sci. 2002;961:183. - PubMed
-
- Sikavitsas V.I. Temenoff J.S. Mikos A.G. Biomaterials and bone mechanotransduction. Biomaterials. 2001;22:2581. - PubMed
-
- Turner C.H. Wang T. Burr D.B. Shear strength and fatigue properties of human cortical bone determined from pure shear tests. Calcif Tissue Int. 2001;69:373. - PubMed
-
- Hillier M.L. Bell L.S. Differentiating human bone from animal bone: a review of histological methods. J Forensic Sci. 2007;52:249. - PubMed
Publication types
MeSH terms
Grants and funding
LinkOut - more resources
Full Text Sources
Other Literature Sources
Miscellaneous