Lipopolysaccharide impairs amyloid β efflux from brain: altered vascular sequestration, cerebrospinal fluid reabsorption, peripheral clearance and transporter function at the blood-brain barrier
- PMID: 22747709
- PMCID: PMC3410805
- DOI: 10.1186/1742-2094-9-150
Lipopolysaccharide impairs amyloid β efflux from brain: altered vascular sequestration, cerebrospinal fluid reabsorption, peripheral clearance and transporter function at the blood-brain barrier
Abstract
Background: Defects in the low density lipoprotein receptor-related protein-1 (LRP-1) and p-glycoprotein (Pgp) clearance of amyloid beta (Aβ) from brain are thought to contribute to Alzheimer's disease (AD). We have recently shown that induction of systemic inflammation by lipopolysaccharide (LPS) results in impaired efflux of Aβ from the brain. The same treatment also impairs Pgp function. Here, our aim is to determine which physiological routes of Aβ clearance are affected following systemic inflammation, including those relying on LRP-1 and Pgp function at the blood-brain barrier.
Methods: CD-1 mice aged between 6 and 8 weeks were treated with 3 intraperitoneal injections of 3 mg/kg LPS at 0, 6, and 24 hours and studied at 28 hours. 125I-Aβ1-42 or 125I-alpha-2-macroglobulin injected into the lateral ventricle of the brain (intracerebroventricular (ICV)) or into the jugular vein (intravenous (IV)) was used to quantify LRP-1-dependent partitioning between the brain vasculature and parenchyma and peripheral clearance, respectively. Disappearance of ICV-injected 14 C-inulin from brain was measured to quantify bulk flow of cerebrospinal fluid (CSF). Brain microvascular protein expression of LRP-1 and Pgp was measured by immunoblotting. Endothelial cell localization of LRP-1 was measured by immunofluorescence microscopy. Oxidative modifications to LRP-1 at the brain microvasculature were measured by immunoprecipitation of LRP-1 followed by immunoblotting for 4-hydroxynonenal and 3-nitrotyrosine.
Results: We found that LPS: caused an LRP-1-dependent redistribution of ICV-injected Aβ from brain parenchyma to brain vasculature and decreased entry into blood; impaired peripheral clearance of IV-injected Aβ; inhibited reabsorption of CSF; did not significantly alter brain microvascular protein levels of LRP-1 or Pgp, or oxidative modifications to LRP-1; and downregulated LRP-1 protein levels and caused LRP-1 mislocalization in cultured brain endothelial cells.
Conclusions: These results suggest that LRP-1 undergoes complex functional regulation following systemic inflammation which may depend on cell type, subcellular location, and post-translational modifications. Our findings that systemic inflammation causes deficits in both Aβ transport and bulk flow like those observed in AD indicate that inflammation could induce and promote the disease.
Figures
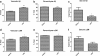
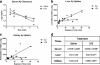
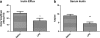
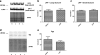
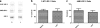
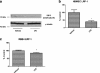
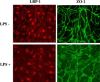
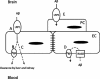
Similar articles
-
Inflammation-induced dysfunction of the low-density lipoprotein receptor-related protein-1 at the blood-brain barrier: protection by the antioxidant N-acetylcysteine.Brain Behav Immun. 2012 Oct;26(7):1085-94. doi: 10.1016/j.bbi.2012.07.003. Epub 2012 Jul 15. Brain Behav Immun. 2012. PMID: 22809665 Free PMC article.
-
Age-Dependent Regulation of the Blood-Brain Barrier Influx/Efflux Equilibrium of Amyloid-β Peptide in a Mouse Model of Alzheimer's Disease (3xTg-AD).J Alzheimers Dis. 2016;49(2):287-300. doi: 10.3233/JAD-150350. J Alzheimers Dis. 2016. PMID: 26484906
-
Amyloid-beta impairs insulin signaling by accelerating autophagy-lysosomal degradation of LRP-1 and IR-β in blood-brain barrier endothelial cells in vitro and in 3XTg-AD mice.Mol Cell Neurosci. 2019 Sep;99:103390. doi: 10.1016/j.mcn.2019.103390. Epub 2019 Jul 2. Mol Cell Neurosci. 2019. PMID: 31276749 Free PMC article.
-
The role of the cell surface LRP and soluble LRP in blood-brain barrier Abeta clearance in Alzheimer's disease.Curr Pharm Des. 2008;14(16):1601-5. doi: 10.2174/138161208784705487. Curr Pharm Des. 2008. PMID: 18673201 Free PMC article. Review.
-
Clearance of amyloid-beta peptide across the blood-brain barrier: implication for therapies in Alzheimer's disease.CNS Neurol Disord Drug Targets. 2009 Mar;8(1):16-30. doi: 10.2174/187152709787601867. CNS Neurol Disord Drug Targets. 2009. PMID: 19275634 Free PMC article. Review.
Cited by
-
Association of Low-Density Lipoprotein Receptor-Related Protein 1 and Its rs1799986 Polymorphism With Mild Cognitive Impairment in Chinese Patients With Type 2 Diabetes.Front Neurosci. 2020 Sep 11;14:743. doi: 10.3389/fnins.2020.00743. eCollection 2020. Front Neurosci. 2020. PMID: 33013281 Free PMC article.
-
Histamine H(3) receptor integrates peripheral inflammatory signals in the neurogenic control of immune responses and autoimmune disease susceptibility.PLoS One. 2013 Jul 22;8(7):e62743. doi: 10.1371/journal.pone.0062743. Print 2013. PLoS One. 2013. PMID: 23894272 Free PMC article.
-
Impaired brain glymphatic flow in experimental hepatic encephalopathy.J Hepatol. 2019 Jan;70(1):40-49. doi: 10.1016/j.jhep.2018.08.021. Epub 2018 Sep 8. J Hepatol. 2019. PMID: 30201461 Free PMC article.
-
Overnutrition Determines LPS Regulation of Mycotoxin Induced Neurotoxicity in Neurodegenerative Diseases.Int J Mol Sci. 2015 Dec 10;16(12):29554-73. doi: 10.3390/ijms161226190. Int J Mol Sci. 2015. PMID: 26690419 Free PMC article. Review.
-
Peripheral Inflammation, Apolipoprotein E4, and Amyloid-β Interact to Induce Cognitive and Cerebrovascular Dysfunction.ASN Neuro. 2017 Jul-Aug;9(4):1759091417719201. doi: 10.1177/1759091417719201. ASN Neuro. 2017. PMID: 28707482 Free PMC article.
References
-
- Thies W, Bleiler L. 2011 Alzheimer's disease facts and figures. Alzheimers Dement. 2011;7:208–244. - PubMed
-
- Zlokovic BV, Deane R, Sagare AP, Bell RD, Winkler EA. Low-density lipoprotein receptor-related protein-1: a serial clearance homeostatic mechanism controlling Alzheimer's amyloid beta-peptide elimination from the brain. J Neurochem. 2010;115:1077–1089. doi: 10.1111/j.1471-4159.2010.07002.x. - DOI - PMC - PubMed
-
- Shibata M, Yamada S, Kumar SR, Calero M, Bading J, Frangione B, Holtzman DM, Miller CA, Strickland DK, Ghiso J, Zlokovic BV. Clearance of Alzheimer's amyloid-ss(1–40) peptide from brain by LDL receptor-related protein-1 at the blood–brain barrier. J Clin Invest. 2000;106:1489–1499. doi: 10.1172/JCI10498. - DOI - PMC - PubMed
-
- Deane R, Wu Z, Sagare A, Davis J, Du Yan S, Hamm K, Xu F, Parisi M, LaRue B, Hu HW, Spijkers P, Guo H, Song X, Lenting PJ, Van Nostrand WE, Zlokovic BV. LRP/amyloid beta-peptide interaction mediates differential brain efflux of Abeta isoforms. Neuron. 2004;43:333–344. doi: 10.1016/j.neuron.2004.07.017. - DOI - PubMed
Publication types
MeSH terms
Substances
Grants and funding
LinkOut - more resources
Full Text Sources
Other Literature Sources
Research Materials
Miscellaneous