Initiation of DNA double strand break repair: signaling and single-stranded resection dictate the choice between homologous recombination, non-homologous end-joining and alternative end-joining
- PMID: 22679557
- PMCID: PMC3365807
Initiation of DNA double strand break repair: signaling and single-stranded resection dictate the choice between homologous recombination, non-homologous end-joining and alternative end-joining
Abstract
A DNA double strand break (DSB) is a highly toxic lesion, which can generate genetic instability and profound genome rearrangements. However, DSBs are required to generate diversity during physiological processes such as meiosis or the establishment of the immune repertoire. Thus, the precise regulation of a complex network of processes is necessary for the maintenance of genomic stability, allowing genetic diversity but protecting against genetic instability and its consequences on oncogenesis. Two main strategies are employed for DSB repair: homologous recombination (HR) and non-homologous end-joining (NHEJ). HR is initiated by single-stranded DNA (ssDNA) resection and requires sequence homology with an intact partner, while NHEJ requires neither resection at initiation nor a homologous partner. Thus, resection is an pivotal step at DSB repair initiation, driving the choice of the DSB repair pathway employed. However, an alternative end-joining (A-EJ) pathway, which is highly mutagenic, has recently been described; A-EJ is initiated by ssDNA resection but does not require a homologous partner. The choice of the appropriate DSB repair system, for instance according the cell cycle stage, is essential for genome stability maintenance. In this context, controlling the initial events of DSB repair is thus an essential step that may be irreversible, and the wrong decision should lead to dramatic consequences. Here, we first present the main DSB repair mechanisms and then discuss the importance of the choice of the appropriate DSB repair pathway according to the cell cycle phase. In a third section, we present the early steps of DSB repair i.e., DSB signaling, chromatin remodeling, and the regulation of ssDNA resection. In the last part, we discuss the competition between the different DSB repair mechanisms. Finally, we conclude with the importance of the fine tuning of this network for genome stability maintenance and for tumor protection in fine.
Keywords: DNA double strand break; Homologous recombination; Non homologous end joining; Resection; alternative end-joining; chromatin remodeling; genetic instability; genome rearrangements.
Figures
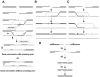
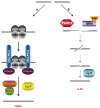
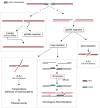
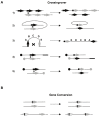
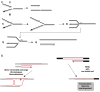
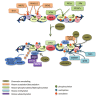
Similar articles
-
Role of the double-strand break repair pathway in the maintenance of genomic stability.Mol Cell Oncol. 2014 Oct 30;2(1):e968020. doi: 10.4161/23723548.2014.968020. eCollection 2015 Jan-Mar. Mol Cell Oncol. 2014. PMID: 27308383 Free PMC article. Review.
-
Analysis of chromatid-break-repair detects a homologous recombination to non-homologous end-joining switch with increasing load of DNA double-strand breaks.Mutat Res Genet Toxicol Environ Mutagen. 2021 Jul;867:503372. doi: 10.1016/j.mrgentox.2021.503372. Epub 2021 Jun 12. Mutat Res Genet Toxicol Environ Mutagen. 2021. PMID: 34266628
-
Alternative end-joining repair pathways are the ultimate backup for abrogated classical non-homologous end-joining and homologous recombination repair: Implications for the formation of chromosome translocations.Mutat Res Genet Toxicol Environ Mutagen. 2015 Nov;793:166-75. doi: 10.1016/j.mrgentox.2015.07.001. Epub 2015 Jul 4. Mutat Res Genet Toxicol Environ Mutagen. 2015. PMID: 26520387 Review.
-
New Facets of DNA Double Strand Break Repair: Radiation Dose as Key Determinant of HR versus c-NHEJ Engagement.Int J Mol Sci. 2023 Oct 6;24(19):14956. doi: 10.3390/ijms241914956. Int J Mol Sci. 2023. PMID: 37834403 Free PMC article. Review.
-
Repair Pathway Choices and Consequences at the Double-Strand Break.Trends Cell Biol. 2016 Jan;26(1):52-64. doi: 10.1016/j.tcb.2015.07.009. Epub 2015 Oct 1. Trends Cell Biol. 2016. PMID: 26437586 Free PMC article. Review.
Cited by
-
Repair of double-strand breaks by end joining.Cold Spring Harb Perspect Biol. 2013 May 1;5(5):a012757. doi: 10.1101/cshperspect.a012757. Cold Spring Harb Perspect Biol. 2013. PMID: 23637284 Free PMC article. Review.
-
High frequency targeted mutagenesis using engineered endonucleases and DNA-end processing enzymes.PLoS One. 2013;8(1):e53217. doi: 10.1371/journal.pone.0053217. Epub 2013 Jan 24. PLoS One. 2013. PMID: 23359797 Free PMC article.
-
Autologous hematopoietic stem cell transplantation in lymphoma patients is associated with a decrease in the double strand break repair capacity of peripheral blood lymphocytes.PLoS One. 2017 Feb 16;12(2):e0171473. doi: 10.1371/journal.pone.0171473. eCollection 2017. PLoS One. 2017. PMID: 28207808 Free PMC article.
-
Vital and Distinct Roles of H2A.Z Isoforms in Hepatocellular Carcinoma.Onco Targets Ther. 2020 May 18;13:4319-4337. doi: 10.2147/OTT.S243823. eCollection 2020. Onco Targets Ther. 2020. PMID: 32547065 Free PMC article.
-
New frontier in regenerative medicine: site-specific gene correction in patient-specific induced pluripotent stem cells.Hum Gene Ther. 2013 Jun;24(6):571-83. doi: 10.1089/hum.2012.251. Hum Gene Ther. 2013. PMID: 23675640 Free PMC article. Review.
References
-
- Bartkova J, Horejsi Z, Koed K, Kramer A, Tort F, Zieger K, Guldberg P, Sehested M, Nesland JM, Lukas C, Orntoft T, Lukas J, Bartek J. DNA damage response as a candidate anti-cancer barrier in early human tumorigenesis. Nature. 2005;434:864–870. - PubMed
-
- Gorgoulis VG, Vassiliou LV, Karakaidos P, Zacharatos P, Kotsinas A, Liloglou T, Venere M, Ditullio RA Jr, Kastrinakis NG, Levy B, Kletsas D, Yoneta A, Herlyn M, Kittas C, Halazonetis TD. Activation of the DNA damage checkpoint and genomic instability in human precancerous lesions. Nature. 2005;434:907–913. - PubMed
-
- Hyrien O. Mechanisms and consequences of replication fork arrest. Biochimie. 2000;82:5–17. - PubMed
LinkOut - more resources
Full Text Sources
Other Literature Sources