Differentiation of multipotent vascular stem cells contributes to vascular diseases
- PMID: 22673902
- PMCID: PMC3538044
- DOI: 10.1038/ncomms1867
Differentiation of multipotent vascular stem cells contributes to vascular diseases
Abstract
It is generally accepted that the de-differentiation of smooth muscle cells, from the contractile to the proliferative/synthetic phenotype, has an important role during vascular remodelling and diseases. Here we provide evidence that challenges this theory. We identify a new type of stem cell in the blood vessel wall, named multipotent vascular stem cells. Multipotent vascular stem cells express markers, including Sox17, Sox10 and S100β, are cloneable, have telomerase activity, and can differentiate into neural cells and mesenchymal stem cell-like cells that subsequently differentiate into smooth muscle cells. On the other hand, we perform lineage tracing with smooth muscle myosin heavy chain as a marker and find that multipotent vascular stem cells and proliferative or synthetic smooth muscle cells do not arise from the de-differentiation of mature smooth muscle cells. In response to vascular injuries, multipotent vascular stem cells, instead of smooth muscle cells, become proliferative, and differentiate into smooth muscle cells and chondrogenic cells, thus contributing to vascular remodelling and neointimal hyperplasia. These findings support a new hypothesis that the differentiation of multipotent vascular stem cells, rather than the de-differentiation of smooth muscle cells, contributes to vascular remodelling and diseases.
Conflict of interest statement
The authors declared no conflict of financial interest.
Figures
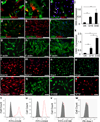
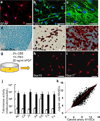
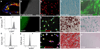
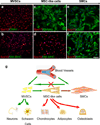
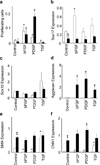
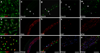
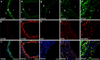
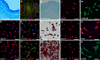
Similar articles
-
Adult vascular smooth muscle cells in culture express neural stem cell markers typical of resident multipotent vascular stem cells.Cell Tissue Res. 2014 Oct;358(1):203-16. doi: 10.1007/s00441-014-1937-2. Epub 2014 Jul 4. Cell Tissue Res. 2014. PMID: 24992927 Free PMC article.
-
Inhibitory role of reactive oxygen species in the differentiation of multipotent vascular stem cells into vascular smooth muscle cells in rats: a novel aspect of traditional culture of rat aortic smooth muscle cells.Cell Tissue Res. 2015 Oct;362(1):97-113. doi: 10.1007/s00441-015-2193-9. Epub 2015 May 29. Cell Tissue Res. 2015. PMID: 26022334
-
Embryonic rat vascular smooth muscle cells revisited - a model for neonatal, neointimal SMC or differentiated vascular stem cells?Vasc Cell. 2014 Mar 15;6(1):6. doi: 10.1186/2045-824X-6-6. Vasc Cell. 2014. PMID: 24628920 Free PMC article.
-
Do Vascular Pericytes Contribute to Neurovasculogenesis in the Central Nervous System as Multipotent Vascular Stem Cells?Stem Cells Dev. 2015 Aug 1;24(15):1730-9. doi: 10.1089/scd.2015.0039. Epub 2015 Jun 3. Stem Cells Dev. 2015. PMID: 25900222 Review.
-
Smooth muscle cell-driven vascular diseases and molecular mechanisms of VSMC plasticity.Cell Signal. 2018 Dec;52:48-64. doi: 10.1016/j.cellsig.2018.08.019. Epub 2018 Aug 30. Cell Signal. 2018. PMID: 30172025 Review.
Cited by
-
Age and vascular aging: an unexplored frontier.Front Cardiovasc Med. 2023 Nov 9;10:1278795. doi: 10.3389/fcvm.2023.1278795. eCollection 2023. Front Cardiovasc Med. 2023. PMID: 38028481 Free PMC article. Review.
-
MicroRNA-30 inhibits neointimal hyperplasia by targeting Ca(2+)/calmodulin-dependent protein kinase IIδ (CaMKIIδ).Sci Rep. 2016 May 20;6:26166. doi: 10.1038/srep26166. Sci Rep. 2016. PMID: 27199283 Free PMC article.
-
S100B is required for maintaining an intermediate state with double-positive Sca-1+ progenitor and vascular smooth muscle cells during neointimal formation.Stem Cell Res Ther. 2019 Sep 23;10(1):294. doi: 10.1186/s13287-019-1400-0. Stem Cell Res Ther. 2019. PMID: 31547879 Free PMC article.
-
Relevance of Oxygen Concentration in Stem Cell Culture for Regenerative Medicine.Int J Mol Sci. 2019 Mar 8;20(5):1195. doi: 10.3390/ijms20051195. Int J Mol Sci. 2019. PMID: 30857245 Free PMC article. Review.
-
Purification and functional assessment of smooth muscle cells derived from mouse embryonic stem cells.J Geriatr Cardiol. 2013 Sep;10(3):272-80. doi: 10.3969/j.issn.1671-5411.2013.03.003. J Geriatr Cardiol. 2013. PMID: 24133516 Free PMC article.
References
-
- Ross R. Atherosclerosis--an inflammatory disease. N Engl J Med. 1999;340:115–126. - PubMed
-
- Libby P. Inflammation in atherosclerosis. Nature. 2002;420:868–874. - PubMed
-
- Hansson GK. Inflammation, atherosclerosis, and coronary artery disease. N Engl J Med. 2005;352:1685–1695. - PubMed
-
- Weissberg PL, Clesham GJ, Bennett MR. Is vascular smooth muscle cell proliferation beneficial? Lancet. 1996;347:305–307. - PubMed