The SMS domain of Trs23p is responsible for the in vitro appearance of the TRAPP I complex in Saccharomyces cerevisiae
- PMID: 22645708
- PMCID: PMC3355973
- DOI: 10.4161/cl.19414
The SMS domain of Trs23p is responsible for the in vitro appearance of the TRAPP I complex in Saccharomyces cerevisiae
Abstract
Saccharomyces cerevisiae transport protein particle (TRAPP) is a family of related multisubunit complexes required for endoplasmic reticulum-to-Golgi transport (TRAPP I), endosome-to-Golgi transport (TRAPP II) or cytosol to vacuole targeting (TRAPP III). To gain insight into the relationship between these complexes, we generated random and targeted mutations in the Trs23p core subunit. Remarkably, at physiological salt concentrations only two peaks (TRAPP I and a high molecular weight peak) are detected in wild-type cells. As the salt was raised, the high molecular weight peak resolved into TRAPP II and III peaks. Deletion of a Saccharomycotina-specific domain of Trs23p resulted in destabilization of TRAPP I but had no effect on TRAPP II or III. This mutation had no observable growth phenotype, normal levels of Ypt1p-directed guanine nucleotide exchange factor activity in vivo and did not display any in vivo nor in vitro blocks in membrane traffic. Biochemical analysis indicated that TRAPP I could be produced from the TRAPP II/III peak in vitro by increasing the salt concentration. Our data suggest that the SMS domain of Trs23p is responsible for the in vitro appearance of TRAPP I in S. cerevisiae. The implications of these findings are discussed.
Figures
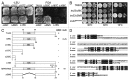
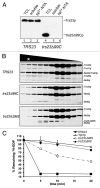
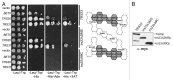
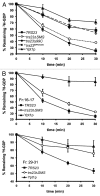
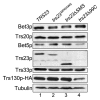
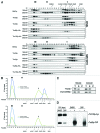
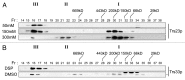
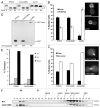
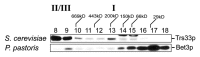
Similar articles
-
TRAPP Complexes in Secretion and Autophagy.Front Cell Dev Biol. 2016 Mar 30;4:20. doi: 10.3389/fcell.2016.00020. eCollection 2016. Front Cell Dev Biol. 2016. PMID: 27066478 Free PMC article. Review.
-
TRAPP stimulates guanine nucleotide exchange on Ypt1p.J Cell Biol. 2000 Oct 16;151(2):289-96. doi: 10.1083/jcb.151.2.289. J Cell Biol. 2000. PMID: 11038176 Free PMC article.
-
Kinetic analysis of the guanine nucleotide exchange activity of TRAPP, a multimeric Ypt1p exchange factor.J Mol Biol. 2009 Jun 5;389(2):275-88. doi: 10.1016/j.jmb.2009.03.068. Epub 2009 Apr 8. J Mol Biol. 2009. PMID: 19361519 Free PMC article.
-
The TRAPP complex is a nucleotide exchanger for Ypt1 and Ypt31/32.Mol Biol Cell. 2000 Dec;11(12):4403-11. doi: 10.1091/mbc.11.12.4403. Mol Biol Cell. 2000. PMID: 11102533 Free PMC article.
-
In sickness and in health: the role of TRAPP and associated proteins in disease.Traffic. 2014 Aug;15(8):803-18. doi: 10.1111/tra.12183. Epub 2014 Jul 1. Traffic. 2014. PMID: 24917561 Review.
Cited by
-
Ypt/Rab GTPases and their TRAPP GEFs at the Golgi.FEBS Lett. 2019 Sep;593(17):2488-2500. doi: 10.1002/1873-3468.13574. Epub 2019 Aug 21. FEBS Lett. 2019. PMID: 31400292 Free PMC article. Review.
-
Characterization of three TRAPPC11 variants suggests a critical role for the extreme carboxy terminus of the protein.Sci Rep. 2019 Oct 1;9(1):14036. doi: 10.1038/s41598-019-50415-6. Sci Rep. 2019. PMID: 31575891 Free PMC article.
-
The TRAPP complexes: discriminating GTPases in context.FEBS Lett. 2023 Mar;597(6):721-733. doi: 10.1002/1873-3468.14557. Epub 2022 Dec 21. FEBS Lett. 2023. PMID: 36481981 Free PMC article. Review.
-
The Role of Secretory Pathways in Candida albicans Pathogenesis.J Fungi (Basel). 2020 Feb 24;6(1):26. doi: 10.3390/jof6010026. J Fungi (Basel). 2020. PMID: 32102426 Free PMC article. Review.
-
TRAPP Complexes in Secretion and Autophagy.Front Cell Dev Biol. 2016 Mar 30;4:20. doi: 10.3389/fcell.2016.00020. eCollection 2016. Front Cell Dev Biol. 2016. PMID: 27066478 Free PMC article. Review.
References
Grants and funding
LinkOut - more resources
Full Text Sources
Molecular Biology Databases
Research Materials