Engineering nano- and microparticles to tune immunity
- PMID: 22641380
- PMCID: PMC3786137
- DOI: 10.1002/adma.201200446
Engineering nano- and microparticles to tune immunity
Abstract
The immune system can be a cure or cause of disease, fulfilling a protective role in attacking cancer or pathogenic microbes but also causing tissue destruction in autoimmune disorders. Thus, therapies aimed to amplify or suppress immune reactions are of great interest. However, the complex regulation of the immune system, coupled with the potential systemic side effects associated with traditional systemic drug therapies, has presented a major hurdle for the development of successful immunotherapies. Recent progress in the design of synthetic micro- and nano-particles that can target drugs, deliver imaging agents, or stimulate immune cells directly through their physical and chemical properties is leading to new approaches to deliver vaccines, promote immune responses against tumors, and suppress autoimmunity. In addition, novel strategies, such as the use of particle-laden immune cells as living targeting agents for drugs, are providing exciting new approaches for immunotherapy. This progress report describes recent advances in the design of micro- and nano-particles for immunotherapies and diagnostics.
Copyright © 2012 WILEY-VCH Verlag GmbH & Co. KGaA, Weinheim.
Figures
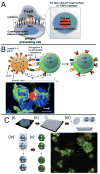
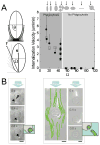
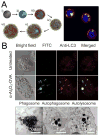
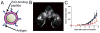
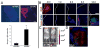
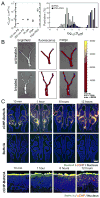
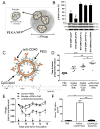
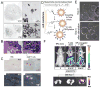
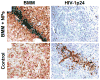
Similar articles
-
Engineering the immune system with particles, step-by-step.Mol Immunol. 2018 Jun;98:25-27. doi: 10.1016/j.molimm.2018.02.015. Epub 2018 Mar 9. Mol Immunol. 2018. PMID: 29526503 Review.
-
Nanoscale artificial antigen presenting cells for cancer immunotherapy.Mol Immunol. 2018 Jun;98:13-18. doi: 10.1016/j.molimm.2018.02.016. Epub 2018 Mar 7. Mol Immunol. 2018. PMID: 29525074 Free PMC article. Review.
-
Biomaterial Strategies for Immunomodulation.Annu Rev Biomed Eng. 2015;17:317-49. doi: 10.1146/annurev-bioeng-071813-104814. Epub 2015 Sep 29. Annu Rev Biomed Eng. 2015. PMID: 26421896 Free PMC article. Review.
-
Nanotechnology-based manipulation of dendritic cells for enhanced immunotherapy strategies.Nanomedicine. 2010 Aug;6(4):523-9. doi: 10.1016/j.nano.2010.01.001. Epub 2010 Jan 18. Nanomedicine. 2010. PMID: 20085824 Review.
-
Polyanhydride Nanoparticles Induce Low Inflammatory Dendritic Cell Activation Resulting in CD8+ T Cell Memory and Delayed Tumor Progression.Int J Nanomedicine. 2020 Sep 7;15:6579-6592. doi: 10.2147/IJN.S261041. eCollection 2020. Int J Nanomedicine. 2020. PMID: 32982219 Free PMC article.
Cited by
-
Modulation of immune responses using adjuvants to facilitate therapeutic vaccination.Immunol Rev. 2020 Jul;296(1):169-190. doi: 10.1111/imr.12889. Epub 2020 Jun 28. Immunol Rev. 2020. PMID: 32594569 Free PMC article. Review.
-
Cell-Based Drug Delivery Systems with Innate Homing Capability as a Novel Nanocarrier Platform.Int J Nanomedicine. 2023 Jan 29;18:509-525. doi: 10.2147/IJN.S394389. eCollection 2023. Int J Nanomedicine. 2023. PMID: 36742991 Free PMC article. Review.
-
Tocopherol Emulsions as Functional Autoantigen Delivery Vehicles Evoke Therapeutic Efficacy in Experimental Autoimmune Encephalomyelitis.Mol Pharm. 2019 Feb 4;16(2):607-617. doi: 10.1021/acs.molpharmaceut.8b00887. Epub 2019 Jan 17. Mol Pharm. 2019. PMID: 30615457 Free PMC article.
-
Effect of nanoparticles on gouty arthritis: a systematic review and meta-analysis.BMC Musculoskelet Disord. 2023 Feb 14;24(1):124. doi: 10.1186/s12891-023-06186-3. BMC Musculoskelet Disord. 2023. PMID: 36788552 Free PMC article.
-
A Nanovaccine Based on Adjuvant Peptide FK-13 and l-Phenylalanine Poly(ester amide) Enhances CD8+ T Cell-Mediated Antitumor Immunity.Adv Sci (Weinh). 2023 Jul;10(20):e2300418. doi: 10.1002/advs.202300418. Epub 2023 May 10. Adv Sci (Weinh). 2023. PMID: 37162249 Free PMC article.
References
-
- Holmgren J, Czerkinsky C. Nat Med. 2005;11:S45. - PubMed
-
- Hodi FS, O’Day SJ, McDermott DF, Weber RW, Sosman JA, Haanen JB, Gonzalez R, Robert C, Schadendorf D, Hassel JC, Akerley W, van den Eertwegh AJ, Lutzky J, Lorigan P, Vaubel JM, Linette GP, Hogg D, Ottensmeier CH, Lebbe C, Peschel C, Quirt I, Clark JI, Wolchok JD, Weber JS, Tian J, Yellin MJ, Nichol GM, Hoos A, Urba WJ. N Engl J Med. 2010;363:711. - PMC - PubMed
Publication types
MeSH terms
Substances
Grants and funding
LinkOut - more resources
Full Text Sources
Other Literature Sources