CAS-1, a C. elegans cyclase-associated protein, is required for sarcomeric actin assembly in striated muscle
- PMID: 22623720
- PMCID: PMC3482317
- DOI: 10.1242/jcs.104950
CAS-1, a C. elegans cyclase-associated protein, is required for sarcomeric actin assembly in striated muscle
Abstract
Assembly of contractile apparatuses in striated muscle requires precisely regulated reorganization of the actin cytoskeletal proteins into sarcomeric organization. Regulation of actin filament dynamics is one of the essential processes of myofibril assembly, but the mechanism of actin regulation in striated muscle is not clearly understood. Actin depolymerizing factor (ADF)/cofilin is a key enhancer of actin filament dynamics in striated muscle in both vertebrates and nematodes. Here, we report that CAS-1, a cyclase-associated protein in Caenorhabditis elegans, promotes ADF/cofilin-dependent actin filament turnover in vitro and is required for sarcomeric actin organization in striated muscle. CAS-1 is predominantly expressed in striated muscle from embryos to adults. In vitro, CAS-1 binds to actin monomers and enhances exchange of actin-bound ATP/ADP even in the presence of UNC-60B, a muscle-specific ADF/cofilin that inhibits the nucleotide exchange. As a result, CAS-1 and UNC-60B cooperatively enhance actin filament turnover. The two proteins also cooperate to shorten actin filaments. A cas-1 mutation is homozygous lethal with defects in sarcomeric actin organization. cas-1-mutant embryos and worms have aggregates of actin in muscle cells, and UNC-60B is mislocalized to the aggregates. These results provide genetic and biochemical evidence that cyclase-associated protein is a critical regulator of sarcomeric actin organization in striated muscle.
Figures
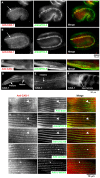
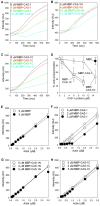
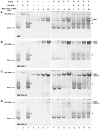
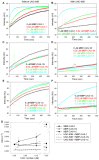
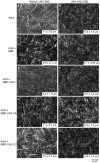
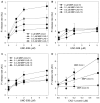
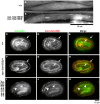
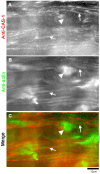
Similar articles
-
Essential role of ADF/cofilin for assembly of contractile actin networks in the C. elegans somatic gonad.J Cell Sci. 2008 Aug 15;121(Pt 16):2662-70. doi: 10.1242/jcs.034215. Epub 2008 Jul 24. J Cell Sci. 2008. PMID: 18653537 Free PMC article.
-
Actin filament disassembling activity of Caenorhabditis elegans actin-interacting protein 1 (UNC-78) is dependent on filament binding by a specific ADF/cofilin isoform.J Cell Sci. 2003 Oct 15;116(Pt 20):4107-18. doi: 10.1242/jcs.00717. Epub 2003 Sep 2. J Cell Sci. 2003. PMID: 12953066
-
The two Caenorhabditis elegans actin-depolymerizing factor/cofilin proteins differently enhance actin filament severing and depolymerization.Biochemistry. 2005 Nov 1;44(43):14238-47. doi: 10.1021/bi050933d. Biochemistry. 2005. PMID: 16245940 Free PMC article.
-
Regulation of structure and function of sarcomeric actin filaments in striated muscle of the nematode Caenorhabditis elegans.Anat Rec (Hoboken). 2014 Sep;297(9):1548-59. doi: 10.1002/ar.22965. Anat Rec (Hoboken). 2014. PMID: 25125169 Free PMC article. Review.
-
Dynamic regulation of sarcomeric actin filaments in striated muscle.Cytoskeleton (Hoboken). 2010 Nov;67(11):677-92. doi: 10.1002/cm.20476. Cytoskeleton (Hoboken). 2010. PMID: 20737540 Free PMC article. Review.
Cited by
-
Mechanism of CAP1-mediated apical actin polymerization in pollen tubes.Proc Natl Acad Sci U S A. 2019 Jun 11;116(24):12084-12093. doi: 10.1073/pnas.1821639116. Epub 2019 May 23. Proc Natl Acad Sci U S A. 2019. PMID: 31123151 Free PMC article.
-
The Cytoskeletal Protein Cyclase-Associated Protein 1 (CAP1) in Breast Cancer: Context-Dependent Roles in Both the Invasiveness and Proliferation of Cancer Cells and Underlying Cell Signals.Int J Mol Sci. 2019 May 30;20(11):2653. doi: 10.3390/ijms20112653. Int J Mol Sci. 2019. PMID: 31151140 Free PMC article. Review.
-
CAP2 in cardiac conduction, sudden cardiac death and eye development.Sci Rep. 2015 Nov 30;5:17256. doi: 10.1038/srep17256. Sci Rep. 2015. PMID: 26616005 Free PMC article.
-
TIAM-1 differentially regulates dendritic and axonal microtubule organization in patterning neuronal development through its multiple domains.PLoS Genet. 2022 Oct 12;18(10):e1010454. doi: 10.1371/journal.pgen.1010454. eCollection 2022 Oct. PLoS Genet. 2022. PMID: 36223408 Free PMC article.
-
ATP-dependent regulation of actin monomer-filament equilibrium by cyclase-associated protein and ADF/cofilin.Biochem J. 2013 Jul 15;453(2):249-59. doi: 10.1042/BJ20130491. Biochem J. 2013. PMID: 23672398 Free PMC article.
References
-
- Agrawal P. B., Greenleaf R. S., Tomczak K. K., Lehtokari V. L., Wallgren–Pettersson C., Wallefeld W., Laing N. G., Darras B. T., Maciver S. K., Dormitzer P. R.et al. (2007). Nemaline myopathy with minicores caused by mutation of the CFL2 gene encoding the skeletal muscle actin-binding protein, cofilin-2. Am. J. Hum. Genet. 80, 162–167 10.1086/510402 - DOI - PMC - PubMed
Publication types
MeSH terms
Substances
Grants and funding
LinkOut - more resources
Full Text Sources
Research Materials