Allostery and binding cooperativity of the catalytic subunit of protein kinase A by NMR spectroscopy and molecular dynamics simulations
- PMID: 22607761
- PMCID: PMC3546502
- DOI: 10.1016/B978-0-12-398312-1.00012-3
Allostery and binding cooperativity of the catalytic subunit of protein kinase A by NMR spectroscopy and molecular dynamics simulations
Abstract
The catalytic subunit of cAMP-dependent protein kinase A (PKA-C) is an exquisite example of a single molecule allosteric enzyme, where classical and modern views of allosteric signaling merge. In this chapter, we describe the mapping of PKA-C conformational dynamics and allosteric signaling in the free and bound states using a combination of NMR spectroscopy and molecular dynamics simulations. We show that ligand binding affects the enzyme's conformational dynamics, shaping the free-energy landscape toward the next stage of the catalytic cycle. While nucleotide and substrate binding enhance the enzyme's conformational entropy and define dynamically committed states, inhibitor binding attenuates the internal dynamics in favor of enthalpic interactions and delineates dynamically quenched states. These studies support a central role of conformational dynamics in many aspects of enzymatic turnover and suggest future avenues for controlling enzymatic function.
Copyright © 2012 Elsevier Inc. All rights reserved.
Figures
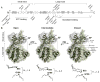
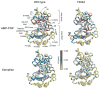
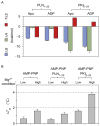
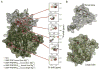
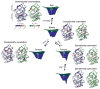
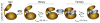
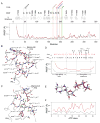
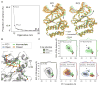
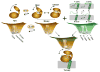
Similar articles
-
Role of conformational entropy in the activity and regulation of the catalytic subunit of protein kinase A.FEBS J. 2013 Nov;280(22):5608-15. doi: 10.1111/febs.12462. Epub 2013 Aug 27. FEBS J. 2013. PMID: 23902454 Free PMC article. Review.
-
The αC-β4 loop controls the allosteric cooperativity between nucleotide and substrate in the catalytic subunit of protein kinase A.Elife. 2024 Jun 24;12:RP91506. doi: 10.7554/eLife.91506. Elife. 2024. PMID: 38913408 Free PMC article.
-
Dynamically committed, uncommitted, and quenched states encoded in protein kinase A revealed by NMR spectroscopy.Proc Natl Acad Sci U S A. 2011 Apr 26;108(17):6969-74. doi: 10.1073/pnas.1102701108. Epub 2011 Apr 6. Proc Natl Acad Sci U S A. 2011. PMID: 21471451 Free PMC article.
-
Water-mediated conformational preselection mechanism in substrate binding cooperativity to protein kinase A.Proc Natl Acad Sci U S A. 2018 Apr 10;115(15):3852-3857. doi: 10.1073/pnas.1720024115. Epub 2018 Mar 26. Proc Natl Acad Sci U S A. 2018. PMID: 29581285 Free PMC article.
-
Allostery in enzyme catalysis.Curr Opin Struct Biol. 2017 Dec;47:123-130. doi: 10.1016/j.sbi.2017.08.002. Epub 2017 Sep 1. Curr Opin Struct Biol. 2017. PMID: 28865247 Review.
Cited by
-
Allosteric regulation of kinase activity in living cells.Elife. 2023 Nov 9;12:RP90574. doi: 10.7554/eLife.90574. Elife. 2023. PMID: 37943025 Free PMC article.
-
Crystal Structures Reveal Hidden Domain Mechanics in Protein Kinase A (PKA).Biology (Basel). 2023 Oct 26;12(11):1370. doi: 10.3390/biology12111370. Biology (Basel). 2023. PMID: 37997969 Free PMC article.
-
A Time-Dependent Quantum Approach to Allostery and a Comparison With Light-Harvesting in Photosynthetic Phenomenon.Front Mol Biosci. 2020 Aug 28;7:156. doi: 10.3389/fmolb.2020.00156. eCollection 2020. Front Mol Biosci. 2020. PMID: 33005625 Free PMC article.
-
Development and Validation of AMBER-FB15-Compatible Force Field Parameters for Phosphorylated Amino Acids.J Phys Chem B. 2021 Nov 4;125(43):11927-11942. doi: 10.1021/acs.jpcb.1c07547. Epub 2021 Oct 20. J Phys Chem B. 2021. PMID: 34668708 Free PMC article.
-
Role of conformational entropy in the activity and regulation of the catalytic subunit of protein kinase A.FEBS J. 2013 Nov;280(22):5608-15. doi: 10.1111/febs.12462. Epub 2013 Aug 27. FEBS J. 2013. PMID: 23902454 Free PMC article. Review.
References
-
- Adams JA. Kinetic and catalytic mechanisms of protein kinases. Chem Rev. 2001;101:2271–2290. - PubMed
-
- Adams JA, Taylor SS. Energetic limits of phosphotransfer in the catalytic subunit of cAMP-dependent protein kinase as measured by viscosity experiments. Biochemistry. 1992;31:8516–8522. - PubMed
-
- Amadei A, Linssen ABM, Berendsen HJC. Essential dynamics of proteins. Proteins. 1993;17:412–425. - PubMed
-
- Baldwin AJ, Kay LE. NMR spectroscopy brings invisible protein states into focus. Nat Chem Biol. 2009;5:808–814. - PubMed
Publication types
MeSH terms
Substances
Grants and funding
- P41 RR002301/RR/NCRR NIH HHS/United States
- T32 DE007288/DE/NIDCR NIH HHS/United States
- S10 RR008438-01/RR/NCRR NIH HHS/United States
- P41 GM066326/GM/NIGMS NIH HHS/United States
- GM 100310/GM/NIGMS NIH HHS/United States
- R01 GM100310/GM/NIGMS NIH HHS/United States
- P41RR02301/RR/NCRR NIH HHS/United States
- P41GM66326/GM/NIGMS NIH HHS/United States
- S10 RR002781/RR/NCRR NIH HHS/United States
- S10 RR008438/RR/NCRR NIH HHS/United States
- T32DE007288/DE/NIDCR NIH HHS/United States
- GM72701/GM/NIGMS NIH HHS/United States
- R01 GM072701/GM/NIGMS NIH HHS/United States
- S10 RR002781-01/RR/NCRR NIH HHS/United States
LinkOut - more resources
Full Text Sources
Other Literature Sources