Down-regulation of GABA(A) receptor via promiscuity with the vasoactive peptide urotensin II receptor. Potential involvement in astrocyte plasticity
- PMID: 22563490
- PMCID: PMC3341351
- DOI: 10.1371/journal.pone.0036319
Down-regulation of GABA(A) receptor via promiscuity with the vasoactive peptide urotensin II receptor. Potential involvement in astrocyte plasticity
Abstract
GABA(A) receptor (GABA(A)R) expression level is inversely correlated with the proliferation rate of astrocytes after stroke or during malignancy of astrocytoma, leading to the hypothesis that GABA(A)R expression/activation may work as a cell proliferation repressor. A number of vasoactive peptides exhibit the potential to modulate astrocyte proliferation, and the question whether these mechanisms may imply alteration in GABA(A)R-mediated functions and/or plasma membrane densities is open. The peptide urotensin II (UII) activates a G protein-coupled receptor named UT, and mediates potent vasoconstriction or vasodilation in mammalian vasculature. We have previously demonstrated that UII activates a PLC/PIPs/Ca(2+) transduction pathway, via both G(q) and G(i/o) proteins and stimulates astrocyte proliferation in culture. It was also shown that UT/G(q)/IP(3) coupling is regulated by the GABA(A)R in rat cultured astrocytes. Here we report that UT and GABA(A)R are co-expressed in cerebellar glial cells from rat brain slices, in human native astrocytes and in glioma cell line, and that UII inhibited the GABAergic activity in rat cultured astrocytes. In CHO cell line co-expressing human UT and combinations of GABA(A)R subunits, UII markedly depressed the GABA current (β(3)γ(2)>α(2)β(3)γ(2)>α(2)β(1)γ(2)). This effect, characterized by a fast short-term inhibition followed by drastic and irreversible run-down, is not relayed by G proteins. The run-down partially involves Ca(2+) and phosphorylation processes, requires dynamin, and results from GABA(A)R internalization. Thus, activation of the vasoactive G protein-coupled receptor UT triggers functional inhibition and endocytosis of GABA(A)R in CHO and human astrocytes, via its receptor C-terminus. This UII-induced disappearance of the repressor activity of GABA(A)R, may play a key role in the initiation of astrocyte proliferation.
Conflict of interest statement
Figures
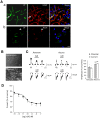
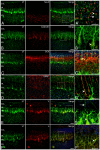
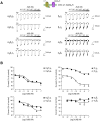
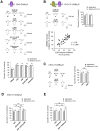
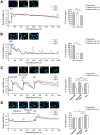
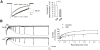
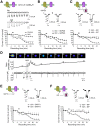
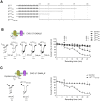
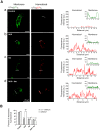
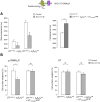
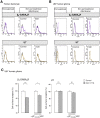
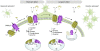
Similar articles
-
Effect of GABA A receptor activation on UT-coupled signaling pathways in rat cortical astrocytes.Peptides. 2008 May;29(5):727-34. doi: 10.1016/j.peptides.2008.01.024. Epub 2008 Feb 15. Peptides. 2008. PMID: 18355946
-
[Orn5]URP acts as a pure antagonist of urotensinergic receptors in rat cortical astrocytes.Peptides. 2008 May;29(5):813-9. doi: 10.1016/j.peptides.2007.10.023. Epub 2007 Nov 6. Peptides. 2008. PMID: 18082287
-
Characterization of urotensin II, distribution of urotensin II, urotensin II-related peptide and UT receptor mRNAs in mouse: evidence of urotensin II at the neuromuscular junction.J Neurochem. 2008 Oct;107(2):361-74. doi: 10.1111/j.1471-4159.2008.05624.x. Epub 2008 Aug 14. J Neurochem. 2008. PMID: 18710417
-
[Research progress of urotensin Ⅱ and urotensin Ⅱ receptor].Yao Xue Xue Bao. 2016 May;51(5):684-9. Yao Xue Xue Bao. 2016. PMID: 29874004 Review. Chinese.
-
International Union of Basic and Clinical Pharmacology. XCII. Urotensin II, urotensin II-related peptide, and their receptor: from structure to function.Pharmacol Rev. 2015;67(1):214-58. doi: 10.1124/pr.114.009480. Pharmacol Rev. 2015. PMID: 25535277 Review.
Cited by
-
Signaling switch of the urotensin II vasosactive peptide GPCR: prototypic chemotaxic mechanism in glioma.Oncogene. 2015 Sep 24;34(39):5080-94. doi: 10.1038/onc.2014.433. Epub 2015 Jan 19. Oncogene. 2015. PMID: 25597409
-
The G Protein-Coupled Receptor UT of the Neuropeptide Urotensin II Displays Structural and Functional Chemokine Features.Front Endocrinol (Lausanne). 2017 Apr 25;8:76. doi: 10.3389/fendo.2017.00076. eCollection 2017. Front Endocrinol (Lausanne). 2017. PMID: 28487672 Free PMC article. Review.
-
Targeting the Urotensin II/UT G Protein-Coupled Receptor to Counteract Angiogenesis and Mesenchymal Hypoxia/Necrosis in Glioblastoma.Front Cell Dev Biol. 2021 Apr 14;9:652544. doi: 10.3389/fcell.2021.652544. eCollection 2021. Front Cell Dev Biol. 2021. PMID: 33937253 Free PMC article.
-
Selenoprotein T Deficiency Leads to Neurodevelopmental Abnormalities and Hyperactive Behavior in Mice.Mol Neurobiol. 2016 Nov;53(9):5818-5832. doi: 10.1007/s12035-015-9505-7. Epub 2015 Oct 26. Mol Neurobiol. 2016. PMID: 26497036
-
Examination of Epigenetic and other Molecular Factors Associated with mda-9/Syntenin Dysregulation in Cancer Through Integrated Analyses of Public Genomic Datasets.Adv Cancer Res. 2015;127:49-121. doi: 10.1016/bs.acr.2015.04.006. Epub 2015 May 23. Adv Cancer Res. 2015. PMID: 26093898 Free PMC article. Review.
References
Publication types
MeSH terms
Substances
LinkOut - more resources
Full Text Sources
Miscellaneous