Prostaglandins in cancer cell adhesion, migration, and invasion
- PMID: 22505934
- PMCID: PMC3299390
- DOI: 10.1155/2012/723419
Prostaglandins in cancer cell adhesion, migration, and invasion
Abstract
Prostaglandins exert a profound influence over the adhesive, migratory, and invasive behavior of cells during the development and progression of cancer. Cyclooxygenase-2 (COX-2) and microsomal prostaglandin E(2) synthase-1 (mPGES-1) are upregulated in inflammation and cancer. This results in the production of prostaglandin E(2) (PGE(2)), which binds to and activates G-protein-coupled prostaglandin E(1-4) receptors (EP(1-4)). Selectively targeting the COX-2/mPGES-1/PGE(2)/EP(1-4) axis of the prostaglandin pathway can reduce the adhesion, migration, invasion, and angiogenesis. Once stimulated by prostaglandins, cadherin adhesive connections between epithelial or endothelial cells are lost. This enables cells to invade through the underlying basement membrane and extracellular matrix (ECM). Interactions with the ECM are mediated by cell surface integrins by "outside-in signaling" through Src and focal adhesion kinase (FAK) and/or "inside-out signaling" through talins and kindlins. Combining the use of COX-2/mPGES-1/PGE(2)/EP(1-4) axis-targeted molecules with those targeting cell surface adhesion receptors or their downstream signaling molecules may enhance cancer therapy.
Figures
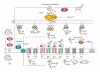
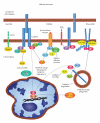
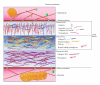
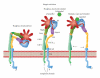
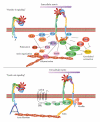
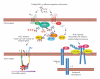
Similar articles
-
Anti-inflammatory role of microsomal prostaglandin E synthase-1 in a model of neuroinflammation.J Biol Chem. 2011 Jan 21;286(3):2331-42. doi: 10.1074/jbc.M110.157362. Epub 2010 Nov 12. J Biol Chem. 2011. PMID: 21075851 Free PMC article.
-
Cyclooxygenase-2, not microsomal prostaglandin E synthase-1, is the mechanism for interleukin-1β-induced prostaglandin E2 production and inhibition of insulin secretion in pancreatic islets.J Biol Chem. 2012 Sep 14;287(38):32246-53. doi: 10.1074/jbc.M112.364612. Epub 2012 Jul 20. J Biol Chem. 2012. PMID: 22822059 Free PMC article.
-
Prostaglandin E₂ receptor EP1-mediated phosphorylation of focal adhesion kinase enhances cell adhesion and migration in hepatocellular carcinoma cells.Int J Oncol. 2013 May;42(5):1833-41. doi: 10.3892/ijo.2013.1859. Epub 2013 Mar 20. Int J Oncol. 2013. PMID: 23525457
-
Membrane prostaglandin E synthase-1: a novel therapeutic target.Pharmacol Rev. 2007 Sep;59(3):207-24. doi: 10.1124/pr.59.3.1. Pharmacol Rev. 2007. PMID: 17878511 Review.
-
Adhesion molecules.Cancer Treat Res. 2002;107:305-29. doi: 10.1007/978-1-4757-3587-1_15. Cancer Treat Res. 2002. PMID: 11775459 Review.
Cited by
-
Identification of AGR2 Gene-Specific Expression Patterns Associated with Epithelial-Mesenchymal Transition.Int J Mol Sci. 2022 Sep 16;23(18):10845. doi: 10.3390/ijms231810845. Int J Mol Sci. 2022. PMID: 36142758 Free PMC article.
-
Vitamin D Signaling in Inflammation and Cancer: Molecular Mechanisms and Therapeutic Implications.Molecules. 2020 Jul 15;25(14):3219. doi: 10.3390/molecules25143219. Molecules. 2020. PMID: 32679655 Free PMC article. Review.
-
Cordycepin inhibits lipopolysaccharide-induced cell migration and invasion in human colorectal carcinoma HCT-116 cells through down-regulation of prostaglandin E2 receptor EP4.BMB Rep. 2018 Oct;51(10):532-537. doi: 10.5483/BMBRep.2018.51.10.120. BMB Rep. 2018. PMID: 30269738 Free PMC article.
-
The Anticancer Potential of Psidium guajava (Guava) Extracts.Life (Basel). 2023 Jan 28;13(2):346. doi: 10.3390/life13020346. Life (Basel). 2023. PMID: 36836712 Free PMC article. Review.
-
Ancient Origins of Cytoskeletal Crosstalk: Spectraplakin-like Proteins Precede the Emergence of Cortical Microtubule Stabilization Complexes as Crosslinkers.Int J Mol Sci. 2022 May 17;23(10):5594. doi: 10.3390/ijms23105594. Int J Mol Sci. 2022. PMID: 35628404 Free PMC article.
References
-
- Shindou H, Shimizu T. Acyl-CoA: lysophospholipid acyltransferases. The Journal of Biological Chemistry. 2009;284(1):1–5. - PubMed
-
- Leslie CC. Regulation of the specific release of arachidonic acid by cytosolic phospholipase A2 . Prostaglandins Leukotrienes and Essential Fatty Acids. 2004;70(4):373–376. - PubMed
Grants and funding
LinkOut - more resources
Full Text Sources
Other Literature Sources
Research Materials
Miscellaneous