Mechanical force alters morphogenetic movements and segmental gene expression patterns during Drosophila embryogenesis
- PMID: 22470437
- PMCID: PMC3310051
- DOI: 10.1371/journal.pone.0033089
Mechanical force alters morphogenetic movements and segmental gene expression patterns during Drosophila embryogenesis
Abstract
The development of an organism is accompanied by various cellular morphogenetic movements, changes in cellular as well as nuclear morphology and transcription programs. Recent evidence suggests that intra and inter-cellular connections mediated by various adhesion proteins contribute to defining nuclear morphology. In addition, three dimensional organization of the cell nucleus regulate the transcription programs. However the link between cellular morphogenetic movements and its coupling to nuclear function in a developmental context is poorly understood. In this paper we use a point perturbation by tissue level laser ablation and sheet perturbation by application of force using magnetic tweezers to alter cellular morphogenetic movements and probe its impact on nuclear morphology and segmental gene expression patterns. Mechanical perturbations during blastoderm stage in a developing Drosophila embryo resulted in localized alterations in nuclear morphology and cellular movement. In addition, global defects in germ-band (GB) extension and retraction are observed when external force is applied during morphogenetic movements, suggesting a long-range physical coupling within the GB layer of cells. Further local application of force resulted in redistribution of non muscle myosin-II in the GB layer. Finally these perturbations lead to altered segmental gene (engrailed) expression patterns later during the development. Our observations suggest that there exists a tight regulation between nuclear morphology and cellular adhesive connections during morphogenetic movement of cells in the embryo. The observed spatial changes in patterning genes, with perturbation, highlight the importance of nuclear integrity to cellular movement in establishing gene expression program in a developmental system.
Conflict of interest statement
Figures
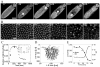
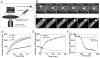
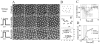
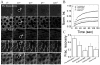
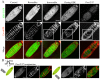
Similar articles
-
Mechanical induction of Twist in the Drosophila foregut/stomodeal primordium.Curr Biol. 2003 Aug 19;13(16):1365-77. doi: 10.1016/s0960-9822(03)00576-1. Curr Biol. 2003. PMID: 12932320
-
Control of germ-band retraction in Drosophila by the zinc-finger protein HINDSIGHT.Development. 1997 Jun;124(11):2129-41. doi: 10.1242/dev.124.11.2129. Development. 1997. PMID: 9187140
-
Amnioserosa development and function in Drosophila embryogenesis: Critical mechanical roles for an extraembryonic tissue.Dev Dyn. 2016 May;245(5):558-68. doi: 10.1002/dvdy.24395. Epub 2016 Mar 15. Dev Dyn. 2016. PMID: 26878336 Review.
-
Integrative approaches to morphogenesis: lessons from dorsal closure.Genesis. 2011 Jul;49(7):522-33. doi: 10.1002/dvg.20704. Epub 2011 Mar 5. Genesis. 2011. PMID: 21162075 Review.
-
Interplay of mechanical deformation and patterned gene expression in developing embryos.Curr Opin Genet Dev. 2004 Aug;14(4):367-74. doi: 10.1016/j.gde.2004.06.005. Curr Opin Genet Dev. 2004. PMID: 15261652 Review.
Cited by
-
A toolbox to explore the mechanics of living embryonic tissues.Semin Cell Dev Biol. 2016 Jul;55:119-30. doi: 10.1016/j.semcdb.2016.03.011. Epub 2016 Apr 6. Semin Cell Dev Biol. 2016. PMID: 27061360 Free PMC article. Review.
-
Bio-Nano-Magnetic Materials for Localized Mechanochemical Stimulation of Cell Growth and Death.Adv Mater. 2016 Jul;28(27):5672-80. doi: 10.1002/adma.201504845. Epub 2016 Jan 18. Adv Mater. 2016. PMID: 26780501 Free PMC article.
-
Significance of 1B and 2B domains in modulating elastic properties of lamin A.Sci Rep. 2016 Jun 15;6:27879. doi: 10.1038/srep27879. Sci Rep. 2016. PMID: 27301336 Free PMC article.
-
Laterally confined growth of cells induces nuclear reprogramming in the absence of exogenous biochemical factors.Proc Natl Acad Sci U S A. 2018 May 22;115(21):E4741-E4750. doi: 10.1073/pnas.1714770115. Epub 2018 May 7. Proc Natl Acad Sci U S A. 2018. PMID: 29735717 Free PMC article.
-
Towards 3D in silico modeling of the sea urchin embryonic development.J Chem Biol. 2013 Sep 13;7(1):17-28. doi: 10.1007/s12154-013-0101-x. J Chem Biol. 2013. PMID: 24386014 Free PMC article. Review.
References
-
- Houchmandzadeh B, Wieschaus E, Leibler S. Establishment of developmental precision and proportions in the early Drosophila embryo. Nature. 2002;415:798–802. - PubMed
-
- Keller R. Cell migration during gastrulation. Curr Opin Cell Biol. 2005;17:533–541. - PubMed
-
- Leptin M. Drosophila gastrulation: from pattern formation to morphogenesis. Annu Rev Cell Dev Biol. 1995;11:189–212. - PubMed
-
- Struhl G, Johnston P, Lawrence PA. Control of Drosophila body pattern by the hunchback morphogen gradient. Cell. 1992;69:237–249. - PubMed
Publication types
MeSH terms
Substances
LinkOut - more resources
Full Text Sources
Molecular Biology Databases