Clustered burst firing in FMR1 premutation hippocampal neurons: amelioration with allopregnanolone
- PMID: 22466801
- PMCID: PMC3373240
- DOI: 10.1093/hmg/dds118
Clustered burst firing in FMR1 premutation hippocampal neurons: amelioration with allopregnanolone
Abstract
Premutation CGG repeat expansions (55-200 CGG repeats; preCGG) within the fragile X mental retardation 1 (FMR1) gene cause fragile X-associated tremor/ataxia syndrome (FXTAS). Defects in neuronal morphology and migration have been described in a preCGG mouse model. Mouse preCGG hippocampal neurons (170 CGG repeats) grown in vitro develop abnormal networks of clustered burst (CB) firing, as assessed by multielectrode array recordings and clustered patterns of spontaneous Ca(2+) oscillations, neither typical of wild-type (WT) neurons. PreCGG neurons have reduced expression of vesicular GABA and glutamate (Glu) transporters (VGAT and VGLUT1, respectively), and preCGG hippocampal astrocytes display a rightward shift on Glu uptake kinetics, compared with WT. These alterations in preCGG astrocytes and neurons are associated with 4- to 8-fold elevated Fmr1 mRNA and occur despite consistent expression of fragile X mental retardation protein levels at ∼50% of WT levels. Abnormal patterns of activity observed in preCGG neurons are pharmacologically mimicked in WT neurons by addition of Glu or the mGluR1/5 agonist, dihydroxyphenylglycine, to the medium, or by inhibition of astrocytic Glu uptake with dl-threo-β-benzyloxyaspartic acid, but not by the ionotropic Glu receptor agonists, α-2-amino-3-(5-methyl-3-oxo-1,2-oxazol-4-yl) propanoic acid or N-methyl-d-aspartic acid. The mGluR1 (7-(hydroxyimino)cyclopropa [b]chromen-1a-carboxylate ethyl ester) or mGluR5 (2-methyl-6-(phenylethynyl)pyridine hydrochloride) antagonists reversed CB firing. Importantly, the acute addition of the neurosteroid allopregnanolone mitigated functional impairments observed in preCGG neurons in a reversible manner. These results demonstrate abnormal mGluR1/5 signaling in preCGG neurons, which is ameliorated by mGluR1/5 antagonists or augmentation of GABA(A) receptor signaling, and identify allopregnanolone as a candidate therapeutic lead.
Figures
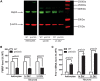
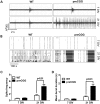
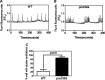
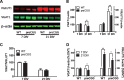
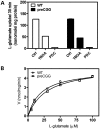
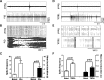
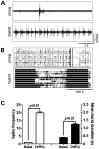
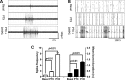
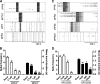
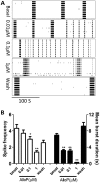
Similar articles
-
Enhanced asynchronous Ca(2+) oscillations associated with impaired glutamate transport in cortical astrocytes expressing Fmr1 gene premutation expansion.J Biol Chem. 2013 May 10;288(19):13831-41. doi: 10.1074/jbc.M112.441055. Epub 2013 Apr 3. J Biol Chem. 2013. PMID: 23553633 Free PMC article.
-
Calcium dysregulation and Cdk5-ATM pathway involved in a mouse model of fragile X-associated tremor/ataxia syndrome.Hum Mol Genet. 2017 Jul 15;26(14):2649-2666. doi: 10.1093/hmg/ddx148. Hum Mol Genet. 2017. PMID: 28444183 Free PMC article.
-
Murine hippocampal neurons expressing Fmr1 gene premutations show early developmental deficits and late degeneration.Hum Mol Genet. 2010 Jan 1;19(1):196-208. doi: 10.1093/hmg/ddp479. Hum Mol Genet. 2010. PMID: 19846466 Free PMC article.
-
Mouse models of fragile X-associated tremor ataxia.J Investig Med. 2009 Dec;57(8):837-41. doi: 10.2310/JIM.0b013e3181af59d6. J Investig Med. 2009. PMID: 19574928 Free PMC article. Review.
-
[FMR1 PREMUTATION CARRIERS - ARE THEY REALLY ASYMPTOMATIC?].Harefuah. 2018 Apr;157(4):241-244. Harefuah. 2018. PMID: 29688643 Review. Hebrew.
Cited by
-
Emerging topics in FXTAS.J Neurodev Disord. 2014;6(1):31. doi: 10.1186/1866-1955-6-31. Epub 2014 Jul 30. J Neurodev Disord. 2014. PMID: 25642984 Free PMC article. Review.
-
Clinical Potential of Neurosteroids for CNS Disorders.Trends Pharmacol Sci. 2016 Jul;37(7):543-561. doi: 10.1016/j.tips.2016.04.003. Epub 2016 May 5. Trends Pharmacol Sci. 2016. PMID: 27156439 Free PMC article. Review.
-
Insight and Recommendations for Fragile X-Premutation-Associated Conditions from the Fifth International Conference on FMR1 Premutation.Cells. 2023 Sep 21;12(18):2330. doi: 10.3390/cells12182330. Cells. 2023. PMID: 37759552 Free PMC article. Review.
-
Associated features in females with an FMR1 premutation.J Neurodev Disord. 2014;6(1):30. doi: 10.1186/1866-1955-6-30. Epub 2014 Jul 30. J Neurodev Disord. 2014. PMID: 25097672 Free PMC article. Review.
-
Open-Label Allopregnanolone Treatment of Men with Fragile X-Associated Tremor/Ataxia Syndrome.Neurotherapeutics. 2017 Oct;14(4):1073-1083. doi: 10.1007/s13311-017-0555-6. Neurotherapeutics. 2017. PMID: 28707277 Free PMC article. Clinical Trial.
References
-
- Jacquemont S., Hagerman R.J., Hagerman P.J., Leehey M.A. Fragile-X syndrome and fragile X-associated tremor/ataxia syndrome: two faces of FMR1. Lancet Neurol. 2007;6:45–55. - PubMed
-
- Brouwer J.R., Severijnen E., de Jong F.H., Hessl D., Hagerman R.J., Oostra B.A., Willemsen R. Altered hypothalamus-pituitary-adrenal gland axis regulation in the expanded CGG-repeat mouse model for fragile X-associated tremor/ataxia syndrome. Psychoneuroendocrinology. 2008;33:863–873. - PMC - PubMed
-
- Fu Y.H., Kuhl D.P., Pizzuti A., Pieretti M., Sutcliffe J.S., Richards S., Verkerk A.J., Holden J.J., Fenwick R.G., Jr, Warren S.T., et al. Variation of the CGG repeat at the fragile X site results in genetic instability: resolution of the Sherman paradox. Cell. 1991;67:1047–1058. - PubMed
Publication types
MeSH terms
Substances
Grants and funding
LinkOut - more resources
Full Text Sources
Other Literature Sources
Medical
Molecular Biology Databases
Miscellaneous