Lessons from mathematically modeling the NF-κB pathway
- PMID: 22435558
- PMCID: PMC3343698
- DOI: 10.1111/j.1600-065X.2011.01092.x
Lessons from mathematically modeling the NF-κB pathway
Abstract
Mathematical modeling has proved to be a critically important approach in the study of many complex networks and dynamic systems in physics, engineering, chemistry, and biology. The nuclear factor κB (NF-κB) system consists of more than 50 proteins and protein complexes and is both a highly networked and dynamic system. To date, mathematical modeling has only addressed a small fraction of the molecular species and their regulation, but when employed in conjunction with experimental analysis has already led to important insights. Here, we provide a personal account of studying how the NF-κB signaling system functions using mathematical descriptions of the molecular mechanisms. We focus on the insights gained about some of the key regulatory components: the control of the steady state, the signaling dynamics, and signaling crosstalk. We also discuss the biological relevance of these regulatory systems properties.
© 2012 John Wiley & Sons A/S.
Figures
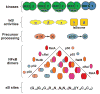
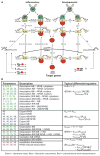
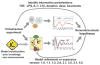
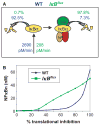
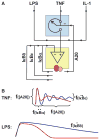
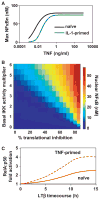
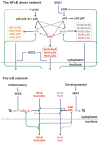
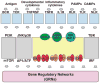
Similar articles
-
Crosstalk via the NF-kappaB signaling system.Cytokine Growth Factor Rev. 2008 Jun-Aug;19(3-4):187-97. doi: 10.1016/j.cytogfr.2008.04.005. Epub 2008 Jun 2. Cytokine Growth Factor Rev. 2008. PMID: 18515173 Free PMC article. Review.
-
A roadmap of constitutive NF-κB activity in Hodgkin lymphoma: Dominant roles of p50 and p52 revealed by genome-wide analyses.Genome Med. 2016 Mar 17;8(1):28. doi: 10.1186/s13073-016-0280-5. Genome Med. 2016. PMID: 26988706 Free PMC article.
-
Generation and activation of multiple dimeric transcription factors within the NF-kappaB signaling system.Mol Cell Biol. 2008 May;28(10):3139-50. doi: 10.1128/MCB.01469-07. Epub 2008 Feb 25. Mol Cell Biol. 2008. PMID: 18299388 Free PMC article.
-
NF-kappaB signalling proteins p50/p105, p52/p100, RelA, and IKKepsilon are over-expressed in oesophageal squamous cell carcinomas.Pathology. 2009;41(7):622-5. doi: 10.3109/00313020903257756. Pathology. 2009. PMID: 20001340
-
[Noncanonical NF-κB pathway and hematological malignancies].Zhongguo Shi Yan Xue Ye Xue Za Zhi. 2010 Aug;18(4):1069-73. Zhongguo Shi Yan Xue Ye Xue Za Zhi. 2010. PMID: 20723331 Review. Chinese.
Cited by
-
Curcumin attenuates renal interstitial fibrosis of obstructive nephropathy by suppressing epithelial-mesenchymal transition through inhibition of the TLR4/NF-кB and PI3K/AKT signalling pathways.Pharm Biol. 2020 Dec;58(1):828-837. doi: 10.1080/13880209.2020.1809462. Pharm Biol. 2020. PMID: 32866059 Free PMC article.
-
Mathematical Models for Immunology: Current State of the Art and Future Research Directions.Bull Math Biol. 2016 Oct;78(10):2091-2134. doi: 10.1007/s11538-016-0214-9. Epub 2016 Oct 6. Bull Math Biol. 2016. PMID: 27714570 Free PMC article. Review.
-
Immune Differentiation Regulator p100 Tunes NF-κB Responses to TNF.Front Immunol. 2019 May 7;10:997. doi: 10.3389/fimmu.2019.00997. eCollection 2019. Front Immunol. 2019. PMID: 31134075 Free PMC article.
-
Considering Abundance, Affinity, and Binding Site Availability in the NF-κB Target Selection Puzzle.Front Immunol. 2019 Mar 29;10:609. doi: 10.3389/fimmu.2019.00609. eCollection 2019. Front Immunol. 2019. PMID: 30984185 Free PMC article. Review.
-
A Quantitative Modular Modeling Approach Reveals the Effects of Different A20 Feedback Implementations for the NF-kB Signaling Dynamics.Front Physiol. 2020 Jul 28;11:896. doi: 10.3389/fphys.2020.00896. eCollection 2020. Front Physiol. 2020. PMID: 32848849 Free PMC article.
References
Publication types
MeSH terms
Substances
Grants and funding
LinkOut - more resources
Full Text Sources