Degradation of MONOCULM 1 by APC/C(TAD1) regulates rice tillering
- PMID: 22434193
- PMCID: PMC3316885
- DOI: 10.1038/ncomms1743
Degradation of MONOCULM 1 by APC/C(TAD1) regulates rice tillering
Abstract
A rice tiller is a specialized grain-bearing branch that contributes greatly to grain yield. The MONOCULM 1 (MOC1) gene is the first identified key regulator controlling rice tiller number; however, the underlying mechanism remains to be elucidated. Here we report a novel rice gene, Tillering and Dwarf 1 (TAD1), which encodes a co-activator of the anaphase-promoting complex (APC/C), a multi-subunit E3 ligase. Although the elucidation of co-activators and individual subunits of plant APC/C involved in regulating plant development have emerged recently, the understanding of whether and how this large cell-cycle machinery controls plant development is still very limited. Our study demonstrates that TAD1 interacts with MOC1, forms a complex with OsAPC10 and functions as a co-activator of APC/C to target MOC1 for degradation in a cell-cycle-dependent manner. Our findings uncovered a new mechanism underlying shoot branching and shed light on the understanding of how the cell-cycle machinery regulates plant architecture.
Figures
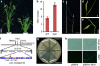
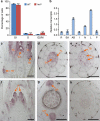
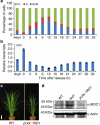
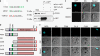
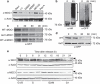
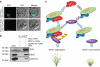
Similar articles
-
Rice APC/C(TE) controls tillering by mediating the degradation of MONOCULM 1.Nat Commun. 2012 Mar 20;3:752. doi: 10.1038/ncomms1716. Nat Commun. 2012. PMID: 22434195 Free PMC article.
-
Potential role of the rice OsCCS52A gene in endoreduplication.Planta. 2012 Feb;235(2):387-97. doi: 10.1007/s00425-011-1515-8. Epub 2011 Sep 17. Planta. 2012. PMID: 21927949
-
DWARF AND LESS TILLERS ON CHROMOSOME 3 promotes tillering in rice by sustaining FLORAL ORGAN NUMBER 1 expression.Plant Physiol. 2024 Oct 1;196(2):1064-1079. doi: 10.1093/plphys/kiae367. Plant Physiol. 2024. PMID: 38996044
-
Tillering and panicle branching genes in rice.Gene. 2014 Mar 1;537(1):1-5. doi: 10.1016/j.gene.2013.11.058. Epub 2013 Dec 15. Gene. 2014. PMID: 24345551 Review.
-
APC-targeted RAA1 degradation mediates the cell cycle and root development in plants.Plant Signal Behav. 2010 Mar;5(3):218-23. doi: 10.4161/psb.5.3.10661. Epub 2010 Mar 14. Plant Signal Behav. 2010. PMID: 20037474 Free PMC article. Review.
Cited by
-
The SnRK2-APC/C(TE) regulatory module mediates the antagonistic action of gibberellic acid and abscisic acid pathways.Nat Commun. 2015 Aug 14;6:7981. doi: 10.1038/ncomms8981. Nat Commun. 2015. PMID: 26272249 Free PMC article.
-
Transcriptomic and Co-Expression Network Profiling of Shoot Apical Meristem Reveal Contrasting Response to Nitrogen Rate between Indica and Japonica Rice Subspecies.Int J Mol Sci. 2019 Nov 25;20(23):5922. doi: 10.3390/ijms20235922. Int J Mol Sci. 2019. PMID: 31775351 Free PMC article.
-
SAMBA controls cell division rate during maize development.Plant Physiol. 2022 Jan 20;188(1):411-424. doi: 10.1093/plphys/kiab514. Plant Physiol. 2022. PMID: 34791456 Free PMC article.
-
Genetic basis controlling rice plant architecture and its modification for breeding.Breed Sci. 2023 Mar;73(1):3-45. doi: 10.1270/jsbbs.22088. Epub 2023 Mar 29. Breed Sci. 2023. PMID: 37168811 Free PMC article.
-
Uncovering the genetic mechanisms regulating panicle architecture in rice with GPWAS and GWAS.BMC Genomics. 2021 Jan 28;22(1):86. doi: 10.1186/s12864-021-07391-x. BMC Genomics. 2021. PMID: 33509071 Free PMC article.
References
Publication types
MeSH terms
Substances
LinkOut - more resources
Full Text Sources