Light-induced retinal degeneration is prevented by zinc, a component in the age-related eye disease study formulation
- PMID: 22385127
- PMCID: PMC4544865
- DOI: 10.1111/j.1751-1097.2012.01134.x
Light-induced retinal degeneration is prevented by zinc, a component in the age-related eye disease study formulation
Abstract
Mineral supplements are often included in multivitamin preparations because of their beneficial effects on metabolism. In this study, we used an animal model of light-induced retinal degeneration to test for photoreceptor cell protection by the essential trace element zinc. Rats were treated with various doses of zinc oxide and then exposed to intense visible light for as long as 8 h. Zinc treatment effectively prevented retinal light damage as determined by rhodopsin and retinal DNA recovery, histology and electrophoretic analysis of DNA damage and oxidized retinal proteins. Zinc oxide was particularly effective when given before light exposure and at doses two- to four-fold higher than recommended by the age-related eye disease study group. Treated rats exhibited higher serum and retinal pigment epithelial zinc levels and an altered retinal gene expression profile. Using an Ingenuity database, 512 genes with known functional annotations were found to be responsive to zinc supplementation, with 45% of these falling into a network related to cellular growth, proliferation, cell cycle and death. Although these data suggest an integrated and extensive regulatory response, zinc induced changes in gene expression also appear to enhance antioxidative capacity in retina and reduce oxidative damage arising from intense light exposure.
© 2012 Wiley Periodicals, Inc. Photochemistry and Photobiology © 2012 The American Society of Photobiology.
Figures
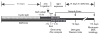
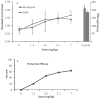
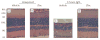
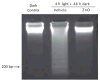
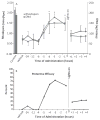
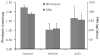
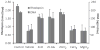
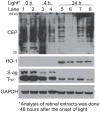
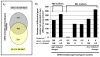
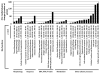
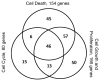
Similar articles
-
Prevention of retinal light damage by zinc oxide combined with rosemary extract.Mol Vis. 2013 Jun 27;19:1433-45. Print 2013. Mol Vis. 2013. PMID: 23825923 Free PMC article.
-
Enhancing the efficacy of AREDS antioxidants in light-induced retinal degeneration.Mol Vis. 2017 Oct 10;23:718-739. eCollection 2017. Mol Vis. 2017. PMID: 29062223 Free PMC article.
-
Light history and age-related changes in retinal light damage.Invest Ophthalmol Vis Sci. 1998 Jun;39(7):1107-16. Invest Ophthalmol Vis Sci. 1998. PMID: 9620069
-
Nanoceria neuroprotective effects in the light-damaged retina: A focus on retinal function and microglia activation.Exp Eye Res. 2019 Nov;188:107797. doi: 10.1016/j.exer.2019.107797. Epub 2019 Sep 11. Exp Eye Res. 2019. PMID: 31520599 Review.
-
Retinal light damage: mechanisms and protection.Prog Retin Eye Res. 2010 Mar;29(2):113-34. doi: 10.1016/j.preteyeres.2009.11.004. Epub 2009 Dec 3. Prog Retin Eye Res. 2010. PMID: 19951742 Free PMC article. Review.
Cited by
-
Multifunctional role of zinc in human health: an update.EXCLI J. 2023 Aug 4;22:809-827. doi: 10.17179/excli2023-6335. eCollection 2023. EXCLI J. 2023. PMID: 37780941 Free PMC article. Review.
-
CEP biomarkers as potential tools for monitoring therapeutics.PLoS One. 2013 Oct 1;8(10):e76325. doi: 10.1371/journal.pone.0076325. eCollection 2013. PLoS One. 2013. PMID: 24098476 Free PMC article.
-
Zinc and diabetic retinopathy.J Diabetes Res. 2013;2013:425854. doi: 10.1155/2013/425854. Epub 2013 Mar 17. J Diabetes Res. 2013. PMID: 23671870 Free PMC article.
-
Prevention of retinal light damage by zinc oxide combined with rosemary extract.Mol Vis. 2013 Jun 27;19:1433-45. Print 2013. Mol Vis. 2013. PMID: 23825923 Free PMC article.
-
Imaging free zinc levels in vivo - what can be learned?Inorganica Chim Acta. 2012 Dec 1;393:12-23. doi: 10.1016/j.ica.2012.06.026. Epub 2012 Jun 25. Inorganica Chim Acta. 2012. PMID: 23180883 Free PMC article.
References
-
- Shahinfar S, Edward DP, Tso MO. A pathological study of photoreceptor cell death in retinal photic injury. Curr Eye Res. 1991;10:47–59. - PubMed
-
- Reme C, Weller M, Szczyensy P, Munz P, Hafezi K, Reinboth J-J, Clausen M. Light-induced apoptosis in the rat retina in vivo. In: Anderson RE, LaVail MM, Hollyfield JG, editors. Degenerative Diseases of the Retina. Plenum Press; New York: 1995. pp. 19–25.
-
- Gordon WC, Casey DM, Lukiw WJ, Bazan NG. DNA damage and repair in light-induced photoreceptor degeneration. Invest Ophthalmol Vis Sci. 2002;43:3511–3521. - PubMed
-
- Noell WK, V, Walker S, Kang BS, Berman S. Retinal damage by light in rats. Invest Ophthalmol. 1966;5:450–473. - PubMed
Publication types
MeSH terms
Substances
Grants and funding
LinkOut - more resources
Full Text Sources