Peroxisome biogenesis and function
- PMID: 22303249
- PMCID: PMC3243405
- DOI: 10.1199/tab.0123
Peroxisome biogenesis and function
Abstract
Peroxisomes are small and single membrane-delimited organelles that execute numerous metabolic reactions and have pivotal roles in plant growth and development. In recent years, forward and reverse genetic studies along with biochemical and cell biological analyses in Arabidopsis have enabled researchers to identify many peroxisome proteins and elucidate their functions. This review focuses on the advances in our understanding of peroxisome biogenesis and metabolism, and further explores the contribution of large-scale analysis, such as in sillco predictions and proteomics, in augmenting our knowledge of peroxisome function In Arabidopsis.
Figures
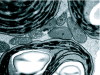
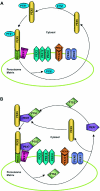
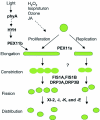
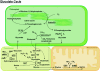
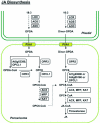
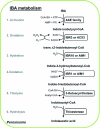
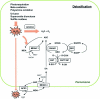
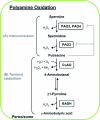
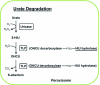
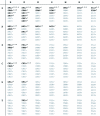
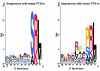
Similar articles
-
Dynamics of Peroxisome Homeostasis and Its Role in Stress Response and Signaling in Plants.Front Plant Sci. 2019 Jun 4;10:705. doi: 10.3389/fpls.2019.00705. eCollection 2019. Front Plant Sci. 2019. PMID: 31214223 Free PMC article. Review.
-
Proteome of Plant Peroxisomes.Subcell Biochem. 2018;89:3-45. doi: 10.1007/978-981-13-2233-4_1. Subcell Biochem. 2018. PMID: 30378017 Review.
-
Novel proteins, putative membrane transporters, and an integrated metabolic network are revealed by quantitative proteomic analysis of Arabidopsis cell culture peroxisomes.Plant Physiol. 2008 Dec;148(4):1809-29. doi: 10.1104/pp.108.129999. Epub 2008 Oct 17. Plant Physiol. 2008. PMID: 18931141 Free PMC article.
-
Plant peroxisomes.Vitam Horm. 2005;72:111-54. doi: 10.1016/S0083-6729(05)72004-5. Vitam Horm. 2005. PMID: 16492470
-
Peroxisomes: versatile organelles with diverse roles in plants.New Phytol. 2020 Feb;225(4):1410-1427. doi: 10.1111/nph.16134. Epub 2019 Sep 26. New Phytol. 2020. PMID: 31442305 Review.
Cited by
-
Iron homeostasis in Arabidopsis thaliana: transcriptomic analyses reveal novel FIT-regulated genes, iron deficiency marker genes and functional gene networks.BMC Plant Biol. 2016 Oct 3;16(1):211. doi: 10.1186/s12870-016-0899-9. BMC Plant Biol. 2016. PMID: 27716045 Free PMC article.
-
Branched-Chain Volatiles in Fruit: A Molecular Perspective.Front Plant Sci. 2022 Jan 27;12:814138. doi: 10.3389/fpls.2021.814138. eCollection 2021. Front Plant Sci. 2022. PMID: 35154212 Free PMC article. Review.
-
Dynamics of Peroxisome Homeostasis and Its Role in Stress Response and Signaling in Plants.Front Plant Sci. 2019 Jun 4;10:705. doi: 10.3389/fpls.2019.00705. eCollection 2019. Front Plant Sci. 2019. PMID: 31214223 Free PMC article. Review.
-
Overexpression of Peroxisome-Localized GmABCA7 Promotes Seed Germination in Arabidopsis thaliana.Int J Mol Sci. 2022 Feb 21;23(4):2389. doi: 10.3390/ijms23042389. Int J Mol Sci. 2022. PMID: 35216505 Free PMC article.
-
Sugar Starvation Disrupts Lipid Breakdown by Inducing Autophagy in Embryonic Axes of Lupin (Lupinus spp.) Germinating Seeds.Int J Mol Sci. 2023 Jul 21;24(14):11773. doi: 10.3390/ijms241411773. Int J Mol Sci. 2023. PMID: 37511532 Free PMC article.
References
-
- Adam Z., Clarke A.K. Cutting edge of chloroplast proteolysis. Trends Plant Sci. 2002;77(1):451–456. - PubMed
-
- Adham A.R., Zolman B.K., Millius A., Bartel B. Mutations in Arabidopsis acyl-CoA oxidase genes reveal distinct and overlapping roles in beta-oxidation. Plant J. 2005;417(1):859–874. - PubMed
-
- Afitlhile M.M., Fukushige H., Nishimura M., Hildebrand D.F. A defect in glyoxysomal fatty acid beta-oxidation reduces jasmonic acid accumulation in Arabidopsis. Plant Physiol. Biochem. 2005;437(1):603–609. - PubMed
-
- Alcazar R., Marco F., Cuevas J.C., Patron M., Ferrando A., Carrasco P., Tiburcio A.F., Altabella T. Involvement of polyamines in plant response to abiotic stress. Biotechnol. Lett. 2006;287(1):1867–1876. - PubMed
-
- Anton M., Passreiter M., Lay D., Thai T.P., Gorgas K., Just W.W. ARF- and coatomer-mediated peroxisomal vesiculation. Cell Biochem. Biophys. 2000;327(1):27–36. Spring. - PubMed
LinkOut - more resources
Full Text Sources