KrasG12D-induced IKK2/β/NF-κB activation by IL-1α and p62 feedforward loops is required for development of pancreatic ductal adenocarcinoma
- PMID: 22264792
- PMCID: PMC3360958
- DOI: 10.1016/j.ccr.2011.12.006
KrasG12D-induced IKK2/β/NF-κB activation by IL-1α and p62 feedforward loops is required for development of pancreatic ductal adenocarcinoma
Abstract
Constitutive Kras and NF-κB activation is identified as signature alterations in pancreatic ductal adenocarcinoma (PDAC). However, how NF-κB is activated in PDAC is not yet understood. Here, we report that pancreas-targeted IKK2/β inactivation inhibited NF-κB activation and PDAC development in Kras(G12D) and Kras(G12D);Ink4a/Arf(F/F) mice, demonstrating a mechanistic link between IKK2/β and Kras(G12D) in PDAC inception. Our findings reveal that Kras(G12D)-activated AP-1 induces IL-1α, which, in turn, activates NF-κB and its target genes IL-1α and p62, to initiate IL-1α/p62 feedforward loops for inducing and sustaining NF-κB activity. Furthermore, IL-1α overexpression correlates with Kras mutation, NF-κB activity, and poor survival in PDAC patients. Therefore, our findings demonstrate the mechanism by which IKK2/β/NF-κB is activated by Kras(G12D) through dual feedforward loops of IL-1α/p62.
Copyright © 2012 Elsevier Inc. All rights reserved.
Figures
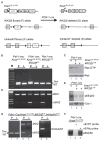
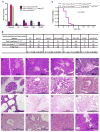
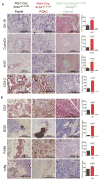
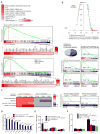
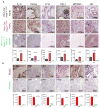
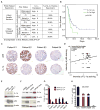
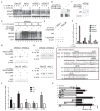
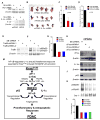
Similar articles
-
Advancement of NF-κB Signaling Pathway: A Novel Target in Pancreatic Cancer.Int J Mol Sci. 2018 Dec 5;19(12):3890. doi: 10.3390/ijms19123890. Int J Mol Sci. 2018. PMID: 30563089 Free PMC article. Review.
-
Loss of Somatostatin Receptor Subtype 2 Promotes Growth of KRAS-Induced Pancreatic Tumors in Mice by Activating PI3K Signaling and Overexpression of CXCL16.Gastroenterology. 2015 Jun;148(7):1452-65. doi: 10.1053/j.gastro.2015.02.009. Epub 2015 Feb 13. Gastroenterology. 2015. PMID: 25683115
-
Ribonucleoprotein HNRNPA2B1 interacts with and regulates oncogenic KRAS in pancreatic ductal adenocarcinoma cells.Gastroenterology. 2014 Oct;147(4):882-892.e8. doi: 10.1053/j.gastro.2014.06.041. Epub 2014 Jul 3. Gastroenterology. 2014. PMID: 24998203
-
Mutant Kras- and p16-regulated NOX4 activation overcomes metabolic checkpoints in development of pancreatic ductal adenocarcinoma.Nat Commun. 2017 Feb 24;8:14437. doi: 10.1038/ncomms14437. Nat Commun. 2017. PMID: 28232723 Free PMC article.
-
KRAS: feeding pancreatic cancer proliferation.Trends Biochem Sci. 2014 Feb;39(2):91-100. doi: 10.1016/j.tibs.2013.12.004. Epub 2014 Jan 2. Trends Biochem Sci. 2014. PMID: 24388967 Free PMC article. Review.
Cited by
-
p62/SQSTM1 enhances breast cancer stem-like properties by stabilizing MYC mRNA.Oncogene. 2017 Jan 19;36(3):304-317. doi: 10.1038/onc.2016.202. Epub 2016 Jun 27. Oncogene. 2017. PMID: 27345399 Free PMC article.
-
TRPV4 activation prevents lipopolysaccharide-induced painful bladder hypersensitivity in rats by regulating immune pathways.Front Immunol. 2022 Dec 22;13:1080302. doi: 10.3389/fimmu.2022.1080302. eCollection 2022. Front Immunol. 2022. PMID: 36618411 Free PMC article.
-
Pancreatic Cancer in Chronic Pancreatitis: Pathogenesis and Diagnostic Approach.Cancers (Basel). 2023 Jan 26;15(3):761. doi: 10.3390/cancers15030761. Cancers (Basel). 2023. PMID: 36765725 Free PMC article. Review.
-
Glucose metabolism and tumour microenvironment in pancreatic cancer: A key link in cancer progression.Front Immunol. 2022 Dec 12;13:1038650. doi: 10.3389/fimmu.2022.1038650. eCollection 2022. Front Immunol. 2022. PMID: 36578477 Free PMC article. Review.
-
Advancement of NF-κB Signaling Pathway: A Novel Target in Pancreatic Cancer.Int J Mol Sci. 2018 Dec 5;19(12):3890. doi: 10.3390/ijms19123890. Int J Mol Sci. 2018. PMID: 30563089 Free PMC article. Review.
References
-
- Brentnall TA, Bronner MP, Byrd DR, Haggitt RC, Kimmey MB. Early diagnosis and treatment of pancreatic dysplasia in patients with a family history of pancreatic cancer. Ann Intern Med. 1999;131:247–255. - PubMed
Publication types
MeSH terms
Substances
Associated data
- Actions
Grants and funding
LinkOut - more resources
Full Text Sources
Other Literature Sources
Medical
Molecular Biology Databases
Miscellaneous