Subsynaptic AMPA receptor distribution is acutely regulated by actin-driven reorganization of the postsynaptic density
- PMID: 22238102
- PMCID: PMC3596168
- DOI: 10.1523/JNEUROSCI.2927-11.2012
Subsynaptic AMPA receptor distribution is acutely regulated by actin-driven reorganization of the postsynaptic density
Abstract
AMPA receptors (AMPARs) mediate synaptic transmission and plasticity during learning, development, and disease. Mechanisms determining subsynaptic receptor position are poorly understood but are key determinants of quantal size. We used a series of live-cell, high-resolution imaging approaches to measure protein organization within single postsynaptic densities in rat hippocampal neurons. By photobleaching receptors in synapse subdomains, we found that most AMPARs do not freely diffuse within the synapse, indicating they are embedded in a matrix that determines their subsynaptic position. However, time lapse analysis revealed that synaptic AMPARs are continuously repositioned in concert with plasticity of this scaffold matrix rather than simply by free diffusion. Using a fluorescence correlation analysis, we found that across the lateral extent of single PSDs, component proteins were differentially distributed, and this distribution was continually adjusted by actin treadmilling. The C-terminal PDZ ligand of GluA1 did not regulate its mobility or distribution in the synapse. However, glutamate receptor activation promoted subsynaptic mobility. Strikingly, subsynaptic immobility of both AMPARs and scaffold molecules remained essentially intact even after loss of actin filaments. We conclude that receptors are actively repositioned at the synapse by treadmilling of the actin cytoskeleton, an influence which is transmitted only indirectly to receptors via the pliable and surprisingly dynamic internal structure of the PSD.
Figures
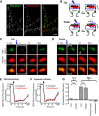
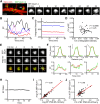
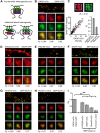
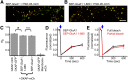
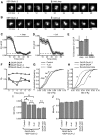
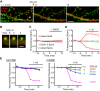
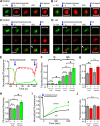
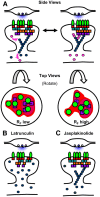
Comment in
-
Microscale AMPAR reorganization and dynamics of the postsynaptic density.J Neurosci. 2012 May 23;32(21):7103-5. doi: 10.1523/JNEUROSCI.1048-12.2012. J Neurosci. 2012. PMID: 22623654 Free PMC article. No abstract available.
Similar articles
-
Microscale AMPAR reorganization and dynamics of the postsynaptic density.J Neurosci. 2012 May 23;32(21):7103-5. doi: 10.1523/JNEUROSCI.1048-12.2012. J Neurosci. 2012. PMID: 22623654 Free PMC article. No abstract available.
-
Lateral organization of the postsynaptic density.Mol Cell Neurosci. 2011 Dec;48(4):321-31. doi: 10.1016/j.mcn.2011.09.001. Epub 2011 Sep 10. Mol Cell Neurosci. 2011. PMID: 21920440 Free PMC article. Review.
-
Protein Crowding within the Postsynaptic Density Can Impede the Escape of Membrane Proteins.J Neurosci. 2016 Apr 13;36(15):4276-95. doi: 10.1523/JNEUROSCI.3154-15.2016. J Neurosci. 2016. PMID: 27076425 Free PMC article.
-
Unified quantitative model of AMPA receptor trafficking at synapses.Proc Natl Acad Sci U S A. 2012 Feb 28;109(9):3522-7. doi: 10.1073/pnas.1109818109. Epub 2012 Feb 13. Proc Natl Acad Sci U S A. 2012. PMID: 22331885 Free PMC article.
-
Regulation of neuronal PKA signaling through AKAP targeting dynamics.Eur J Cell Biol. 2006 Jul;85(7):627-33. doi: 10.1016/j.ejcb.2006.01.010. Epub 2006 Feb 28. Eur J Cell Biol. 2006. PMID: 16504338 Review.
Cited by
-
Nanoscale scaffolding domains within the postsynaptic density concentrate synaptic AMPA receptors.Neuron. 2013 May 22;78(4):615-22. doi: 10.1016/j.neuron.2013.03.009. Neuron. 2013. PMID: 23719161 Free PMC article.
-
Diffusion dynamics of synaptic molecules during inhibitory postsynaptic plasticity.Front Cell Neurosci. 2014 Sep 23;8:300. doi: 10.3389/fncel.2014.00300. eCollection 2014. Front Cell Neurosci. 2014. PMID: 25294987 Free PMC article. Review.
-
Dynamic imaging of AMPA receptor trafficking in vitro and in vivo.Curr Opin Neurobiol. 2017 Aug;45:51-58. doi: 10.1016/j.conb.2017.03.008. Epub 2017 Apr 12. Curr Opin Neurobiol. 2017. PMID: 28411409 Free PMC article. Review.
-
Transcellular Nanoalignment of Synaptic Function.Neuron. 2017 Nov 1;96(3):680-696. doi: 10.1016/j.neuron.2017.10.006. Neuron. 2017. PMID: 29096080 Free PMC article. Review.
-
Abl2:Cortactin Interactions Regulate Dendritic Spine Stability via Control of a Stable Filamentous Actin Pool.J Neurosci. 2021 Apr 7;41(14):3068-3081. doi: 10.1523/JNEUROSCI.2472-20.2021. Epub 2021 Feb 23. J Neurosci. 2021. PMID: 33622779 Free PMC article.
References
-
- Ackermann M, Matus A. Activity-induced targeting of profilin and stabilization of dendritic spine morphology. Nat Neurosci. 2003;6:1194–1200. - PubMed
Publication types
MeSH terms
Substances
Grants and funding
- T32 GM008181/GM/NIGMS NIH HHS/United States
- F31-MH086177/MH/NIMH NIH HHS/United States
- T32GM008181/GM/NIGMS NIH HHS/United States
- R01 MH080046-04/MH/NIMH NIH HHS/United States
- R01 MH080046-02/MH/NIMH NIH HHS/United States
- F31 MH086177/MH/NIMH NIH HHS/United States
- R01 MH080046-03/MH/NIMH NIH HHS/United States
- R01-MH080046/MH/NIMH NIH HHS/United States
- S10 RR024548/RR/NCRR NIH HHS/United States
- R01 MH080046-01/MH/NIMH NIH HHS/United States
- R01 MH080046-05/MH/NIMH NIH HHS/United States
- R37 MH080046/MH/NIMH NIH HHS/United States
- R01 MH080046/MH/NIMH NIH HHS/United States
LinkOut - more resources
Full Text Sources
Other Literature Sources