Parietofrontal integrity determines neural modulation associated with grasping imagery after stroke
- PMID: 22232595
- PMCID: PMC3286199
- DOI: 10.1093/brain/awr331
Parietofrontal integrity determines neural modulation associated with grasping imagery after stroke
Abstract
Chronic stroke patients with heterogeneous lesions, but no direct damage to the primary sensorimotor cortex, are capable of longitudinally acquiring the ability to modulate sensorimotor rhythms using grasping imagery of the affected hand. Volitional modulation of neural activity can be used to drive grasping functions of the paralyzed hand through a brain-computer interface. The neural substrates underlying this skill are not known. Here, we investigated the impact of individual patient's lesion pathology on functional and structural network integrity related to this volitional skill. Magnetoencephalography data acquired throughout training was used to derive functional networks. Structural network models and local estimates of extralesional white matter microstructure were constructed using T(1)-weighted and diffusion-weighted magnetic resonance imaging data. We employed a graph theoretical approach to characterize emergent properties of distributed interactions between nodal brain regions of these networks. We report that interindividual variability in patients' lesions led to differential impairment of functional and structural network characteristics related to successful post-training sensorimotor rhythm modulation skill. Patients displaying greater magnetoencephalography global cost-efficiency, a measure of information integration within the distributed functional network, achieved greater levels of skill. Analysis of lesion damage to structural network connectivity revealed that the impact on nodal betweenness centrality of the ipsilesional primary motor cortex, a measure that characterizes the importance of a brain region for integrating visuomotor information between frontal and parietal cortical regions and related thalamic nuclei, correlated with skill. Edge betweenness centrality, an analogous measure, which assesses the role of specific white matter fibre pathways in network integration, showed a similar relationship between skill and a portion of the ipsilesional superior longitudinal fascicle connecting premotor and posterior parietal visuomotor regions known to be crucially involved in normal grasping behaviour. Finally, estimated white matter microstructure integrity in regions of the contralesional superior longitudinal fascicle adjacent to primary sensorimotor and posterior parietal cortex, as well as grey matter volume co-localized to these specific regions, positively correlated with sensorimotor rhythm modulation leading to successful brain-computer interface control. Thus, volitional modulation of ipsilesional neural activity leading to control of paralyzed hand grasping function through a brain-computer interface after longitudinal training relies on structural and functional connectivity in both ipsilesional and contralesional parietofrontal pathways involved in visuomotor information processing. Extant integrity of this structural network may serve as a future predictor of response to longitudinal therapeutic interventions geared towards training sensorimotor rhythms in the lesioned brain, secondarily improving grasping function through brain-computer interface applications.
Figures
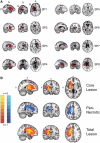
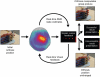
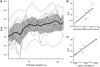
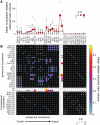
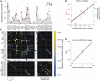
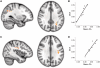
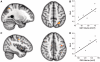
Similar articles
-
Parietofrontal network upregulation after motor stroke.Neuroimage Clin. 2018 Mar 7;18:720-729. doi: 10.1016/j.nicl.2018.03.006. eCollection 2018. Neuroimage Clin. 2018. PMID: 29876261 Free PMC article.
-
Parietofrontal motor pathways and their association with motor function after stroke.Brain. 2015 Jul;138(Pt 7):1949-60. doi: 10.1093/brain/awv100. Epub 2015 May 1. Brain. 2015. PMID: 25935722
-
Frontoparietal Structural Network Disconnections Correlate With Outcome After a Severe Stroke.Hum Brain Mapp. 2024 Nov;45(16):e70060. doi: 10.1002/hbm.70060. Hum Brain Mapp. 2024. PMID: 39487651 Free PMC article.
-
Contribution of transcranial magnetic stimulation in assessing parietofrontal connectivity during gesture production in healthy individuals and brain-injured patients.Neurophysiol Clin. 2019 Apr;49(2):115-123. doi: 10.1016/j.neucli.2018.12.005. Epub 2018 Dec 29. Neurophysiol Clin. 2019. PMID: 30600138 Review.
-
Integrated technology for evaluation of brain function and neural plasticity.Phys Med Rehabil Clin N Am. 2004 Feb;15(1):263-306. doi: 10.1016/s1047-9651(03)00124-4. Phys Med Rehabil Clin N Am. 2004. PMID: 15029909 Review.
Cited by
-
A Systematic Review of the Effects of EEG Neurofeedback on Patients with Schizophrenia.J Pers Med. 2024 Jul 18;14(7):763. doi: 10.3390/jpm14070763. J Pers Med. 2024. PMID: 39064017 Free PMC article. Review.
-
Tasked-Based Functional Brain Connectivity in Multisensory Control of Wrist Movement After Stroke.Front Neurol. 2019 Jun 13;10:609. doi: 10.3389/fneur.2019.00609. eCollection 2019. Front Neurol. 2019. PMID: 31263444 Free PMC article.
-
Myoelectric Computer Interface Training for Reducing Co-Activation and Enhancing Arm Movement in Chronic Stroke Survivors: A Randomized Trial.Neurorehabil Neural Repair. 2019 Apr;33(4):284-295. doi: 10.1177/1545968319834903. Epub 2019 Mar 19. Neurorehabil Neural Repair. 2019. PMID: 30888251 Free PMC article. Clinical Trial.
-
Reducing Abnormal Muscle Coactivation After Stroke Using a Myoelectric-Computer Interface: A Pilot Study.Neurorehabil Neural Repair. 2014 Jun;28(5):443-51. doi: 10.1177/1545968313517751. Epub 2013 Dec 27. Neurorehabil Neural Repair. 2014. PMID: 24376069 Free PMC article.
-
Reinforcement learning for adaptive threshold control of restorative brain-computer interfaces: a Bayesian simulation.Front Neurosci. 2015 Feb 12;9:36. doi: 10.3389/fnins.2015.00036. eCollection 2015. Front Neurosci. 2015. PMID: 25729347 Free PMC article.
References
-
- Ang KK, Guan C, Chua KS, Ang BT, Kuah C, Wang C, et al. Clinical study of neurorehabilitation in stroke using EEG-based motor imagery brain-computer interface with robotic feedback. Conf Proc IEEE Eng Med Biol Soc. 2010;2010:5549–52. - PubMed
-
- Bai O, Lin P, Vorbach S, Floeter MK, Hattori N, Hallett M. A high performance sensorimotor beta rhythm-based brain-computer interface associated with human natural motor behavior. J Neural Eng. 2008;5:24–35. - PubMed
Publication types
MeSH terms
Grants and funding
LinkOut - more resources
Full Text Sources
Medical