SMRT analysis of MTOC and nuclear positioning reveals the role of EB1 and LIC1 in single-cell polarization
- PMID: 22193958
- PMCID: PMC3258110
- DOI: 10.1242/jcs.091231
SMRT analysis of MTOC and nuclear positioning reveals the role of EB1 and LIC1 in single-cell polarization
Abstract
In several migratory cells, the microtubule-organizing center (MTOC) is repositioned between the leading edge and nucleus, creating a polarized morphology. Although our understanding of polarization has progressed as a result of various scratch-wound and cell migration studies, variations in culture conditions required for such assays have prevented a unified understanding of the intricacies of MTOC and nucleus positioning that result in cell polarization. Here, we employ a new SMRT (for sparse, monolayer, round, triangular) analysis that uses a universal coordinate system based on cell centroid to examine the pathways regulating MTOC and nuclear positions in cells plated in a variety of conditions. We find that MTOC and nucleus positioning are crucially and independently affected by cell shape and confluence; MTOC off-centering correlates with the polarization of single cells; acto-myosin contractility and microtubule dynamics are required for single-cell polarization; and end binding protein 1 and light intermediate chain 1, but not Par3 and light intermediate chain 2, are required for single-cell polarization and directional cell motility. Using various cellular geometries and conditions, we implement a systematic and reproducible approach to identify regulators of MTOC and nucleus positioning that depend on extracellular guidance cues.
Figures
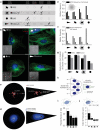
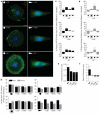
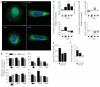
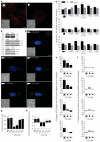
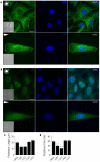
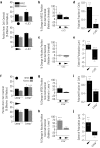
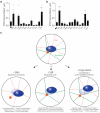
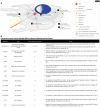
Similar articles
-
Nuclear movement regulated by Cdc42, MRCK, myosin, and actin flow establishes MTOC polarization in migrating cells.Cell. 2005 May 6;121(3):451-63. doi: 10.1016/j.cell.2005.02.022. Cell. 2005. PMID: 15882626
-
Microtubule involvement in NIH 3T3 Golgi and MTOC polarity establishment.J Cell Sci. 2003 Feb 15;116(Pt 4):743-56. doi: 10.1242/jcs.00288. J Cell Sci. 2003. PMID: 12538774
-
Cdc42, dynein, and dynactin regulate MTOC reorientation independent of Rho-regulated microtubule stabilization.Curr Biol. 2001 Oct 2;11(19):1536-41. doi: 10.1016/s0960-9822(01)00475-4. Curr Biol. 2001. PMID: 11591323
-
Mechanisms of nuclear positioning.J Cell Sci. 1998 Aug;111 ( Pt 16):2283-95. doi: 10.1242/jcs.111.16.2283. J Cell Sci. 1998. PMID: 9683624 Review.
-
Microtubule network asymmetry in motile cells: role of Golgi-derived array.Cell Cycle. 2009 Jul 15;8(14):2168-74. doi: 10.4161/cc.8.14.9074. Epub 2009 Jul 20. Cell Cycle. 2009. PMID: 19556895 Free PMC article. Review.
Cited by
-
Force-induced changes in subnuclear movement and rheology.Biophys J. 2012 Dec 19;103(12):2423-31. doi: 10.1016/j.bpj.2012.10.039. Epub 2012 Dec 18. Biophys J. 2012. PMID: 23260044 Free PMC article.
-
Tight coupling between nucleus and cell migration through the perinuclear actin cap.J Cell Sci. 2014 Jun 1;127(Pt 11):2528-41. doi: 10.1242/jcs.144345. Epub 2014 Mar 17. J Cell Sci. 2014. PMID: 24639463 Free PMC article.
-
Enucleated cells reveal differential roles of the nucleus in cell migration, polarity, and mechanotransduction.J Cell Biol. 2018 Mar 5;217(3):895-914. doi: 10.1083/jcb.201706097. Epub 2018 Jan 19. J Cell Biol. 2018. PMID: 29351995 Free PMC article.
-
Microtubule-organizing center-mediated nuclear polarity in various normal and neoplastic human tissues.Virchows Arch. 2015 Jun;466(6):625-35. doi: 10.1007/s00428-015-1744-5. Epub 2015 Mar 6. Virchows Arch. 2015. PMID: 25742907
-
Focal adhesion size uniquely predicts cell migration.FASEB J. 2013 Apr;27(4):1351-61. doi: 10.1096/fj.12-220160. Epub 2012 Dec 19. FASEB J. 2013. PMID: 23254340 Free PMC article.
References
-
- Bornens M. (2008). Organelle positioning and cell polarity. Nat. Rev. Mol. Cell Biol. 9, 874-886 - PubMed
Publication types
MeSH terms
Substances
Grants and funding
LinkOut - more resources
Full Text Sources
Research Materials