The ankyrin repeats and DHHC S-acyl transferase domain of AKR1 act independently to regulate switching from vegetative to mating states in yeast
- PMID: 22174902
- PMCID: PMC3234281
- DOI: 10.1371/journal.pone.0028799
The ankyrin repeats and DHHC S-acyl transferase domain of AKR1 act independently to regulate switching from vegetative to mating states in yeast
Abstract
Signal transduction from G-protein coupled receptors to MAPK cascades through heterotrimeric G-proteins has been described for many eukaryotic systems. One of the best-characterised examples is the yeast pheromone response pathway, which is negatively regulated by AKR1. AKR1-like proteins are present in all eukaryotes and contain a DHHC domain and six ankyrin repeats. Whilst the DHHC domain dependant S-acyl transferase (palmitoyl transferase) function of AKR1 is well documented it is not known whether the ankyrin repeats are also required for this activity. Here we show that the ankyrin repeats of AKR1 are required for full suppression of the yeast pheromone response pathway, by sequestration of the Gβγ dimer, and act independently of AKR1 S-acylation function. Importantly, the functions provided by the AKR1 ankyrin repeats and DHHC domain are not required on the same molecule to fully restore WT phenotypes and function. We also show that AKR1 molecules are S-acylated at locations other than the DHHC cysteine, increasing the abundance of AKR1 in the cell. Our results have important consequences for studies of AKR1 function, including recent attempts to characterise S-acylation enzymology and kinetics. Proteins similar to AKR1 are found in all eukaryotes and our results have broad implications for future work on these proteins and the control of switching between Gβγ regulated pathways.
Conflict of interest statement
Figures
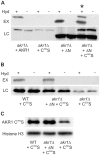
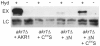
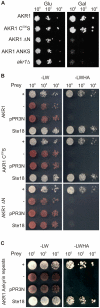
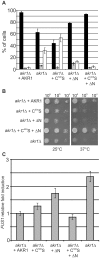
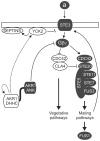
Similar articles
-
Transmembrane topology of the protein palmitoyl transferase Akr1.J Biol Chem. 2005 Mar 18;280(11):10156-63. doi: 10.1074/jbc.M411946200. Epub 2005 Jan 4. J Biol Chem. 2005. PMID: 15632165
-
The ankyrin repeat-containing protein Akr1p is required for the endocytosis of yeast pheromone receptors.Mol Biol Cell. 1997 Jul;8(7):1317-27. doi: 10.1091/mbc.8.7.1317. Mol Biol Cell. 1997. PMID: 9243510 Free PMC article.
-
Interactions between the ankyrin repeat-containing protein Akr1p and the pheromone response pathway in Saccharomyces cerevisiae.Mol Cell Biol. 1996 Jan;16(1):168-78. doi: 10.1128/MCB.16.1.168. Mol Cell Biol. 1996. PMID: 8524293 Free PMC article.
-
On the mechanism of protein palmitoylation.EMBO Rep. 2004 Nov;5(11):1053-7. doi: 10.1038/sj.embor.7400277. EMBO Rep. 2004. PMID: 15520806 Free PMC article. Review.
-
Protein palmitoylation by a family of DHHC protein S-acyltransferases.J Lipid Res. 2006 Jun;47(6):1118-27. doi: 10.1194/jlr.R600007-JLR200. Epub 2006 Apr 1. J Lipid Res. 2006. PMID: 16582420 Review.
Cited by
-
Identification of a Novel Sequence Motif Recognized by the Ankyrin Repeat Domain of zDHHC17/13 S-Acyltransferases.J Biol Chem. 2015 Sep 4;290(36):21939-50. doi: 10.1074/jbc.M115.657668. Epub 2015 Jul 21. J Biol Chem. 2015. PMID: 26198635 Free PMC article.
-
The Cysteine-rich Domain of the DHHC3 Palmitoyltransferase Is Palmitoylated and Contains Tightly Bound Zinc.J Biol Chem. 2015 Dec 4;290(49):29259-69. doi: 10.1074/jbc.M115.691147. Epub 2015 Oct 20. J Biol Chem. 2015. PMID: 26487721 Free PMC article.
-
Genomics and localization of the Arabidopsis DHHC-cysteine-rich domain S-acyltransferase protein family.Plant Physiol. 2012 Nov;160(3):1597-612. doi: 10.1104/pp.112.203968. Epub 2012 Sep 11. Plant Physiol. 2012. PMID: 22968831 Free PMC article.
-
Palmitoylation of the Cysteine Residue in the DHHC Motif of a Palmitoyl Transferase Mediates Ca2+ Homeostasis in Aspergillus.PLoS Genet. 2016 Apr 8;12(4):e1005977. doi: 10.1371/journal.pgen.1005977. eCollection 2016 Apr. PLoS Genet. 2016. PMID: 27058039 Free PMC article.
-
Polyketide synthesis genes associated with toxin production in two species of Gambierdiscus (Dinophyceae).BMC Genomics. 2015 May 28;16(1):410. doi: 10.1186/s12864-015-1625-y. BMC Genomics. 2015. PMID: 26016672 Free PMC article.
References
Publication types
MeSH terms
Substances
Grants and funding
LinkOut - more resources
Full Text Sources
Molecular Biology Databases