Cooperative assembly and dynamic disassembly of MDA5 filaments for viral dsRNA recognition
- PMID: 22160685
- PMCID: PMC3248507
- DOI: 10.1073/pnas.1113651108
Cooperative assembly and dynamic disassembly of MDA5 filaments for viral dsRNA recognition
Abstract
MDA5, an RIG-I-like helicase, is a conserved cytoplasmic viral RNA sensor, which recognizes dsRNA from a wide-range of viruses in a length-dependent manner. It has been proposed that MDA5 forms higher-order structures upon viral dsRNA recognition or during antiviral signaling, however the organization and nature of this proposed oligomeric state is unknown. We report here that MDA5 cooperatively assembles into a filamentous oligomer composed of a repeating segmental arrangement of MDA5 dimers along the length of dsRNA. Binding of MDA5 to dsRNA stimulates its ATP hydrolysis activity with little coordination between neighboring molecules within a filament. Individual ATP hydrolysis in turn renders an intrinsic kinetic instability to the MDA5 filament, triggering dissociation of MDA5 from dsRNA at a rate inversely proportional to the filament length. These results suggest a previously unrecognized role of ATP hydrolysis in control of filament assembly and disassembly processes, thereby autoregulating the interaction of MDA5 with dsRNA, and provides a potential basis for dsRNA length-dependent antiviral signaling.
Conflict of interest statement
The authors declare no conflict of interest.
Figures
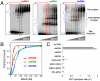
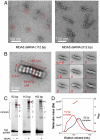
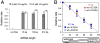
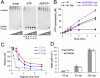
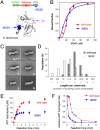
Similar articles
-
LGP2 synergy with MDA5 in RLR-mediated RNA recognition and antiviral signaling.Cytokine. 2015 Aug;74(2):198-206. doi: 10.1016/j.cyto.2015.02.010. Epub 2015 Mar 18. Cytokine. 2015. PMID: 25794939 Free PMC article. Review.
-
Kinetic mechanism for viral dsRNA length discrimination by MDA5 filaments.Proc Natl Acad Sci U S A. 2012 Dec 4;109(49):E3340-9. doi: 10.1073/pnas.1208618109. Epub 2012 Nov 5. Proc Natl Acad Sci U S A. 2012. PMID: 23129641 Free PMC article.
-
Contrasting functions of ATP hydrolysis by MDA5 and LGP2 in viral RNA sensing.J Biol Chem. 2024 Mar;300(3):105711. doi: 10.1016/j.jbc.2024.105711. Epub 2024 Feb 1. J Biol Chem. 2024. PMID: 38309507 Free PMC article.
-
ATP-dependent effector-like functions of RIG-I-like receptors.Mol Cell. 2015 May 7;58(3):541-548. doi: 10.1016/j.molcel.2015.03.014. Epub 2015 Apr 16. Mol Cell. 2015. PMID: 25891073 Free PMC article.
-
MDA5-filament, dynamics and disease.Curr Opin Virol. 2015 Jun;12:20-5. doi: 10.1016/j.coviro.2015.01.011. Epub 2015 Feb 9. Curr Opin Virol. 2015. PMID: 25676875 Free PMC article. Review.
Cited by
-
[New aspects on the pathogenesis of myositis].Z Rheumatol. 2013 Apr;72(3):209-19. doi: 10.1007/s00393-012-1078-5. Z Rheumatol. 2013. PMID: 23515563 German.
-
Assembly-driven activation of the AIM2 foreign-dsDNA sensor provides a polymerization template for downstream ASC.Nat Commun. 2015 Jul 22;6:7827. doi: 10.1038/ncomms8827. Nat Commun. 2015. PMID: 26197926 Free PMC article.
-
Enter at your own risk: how enteroviruses navigate the dangerous world of pattern recognition receptor signaling.Cytokine. 2013 Sep;63(3):230-6. doi: 10.1016/j.cyto.2013.05.007. Epub 2013 Jun 10. Cytokine. 2013. PMID: 23764548 Free PMC article. Review.
-
Trafficking of mitochondrial double-stranded RNA from mitochondria to the cytosol.Life Sci Alliance. 2024 Jul 2;7(9):e202302396. doi: 10.26508/lsa.202302396. Print 2024 Sep. Life Sci Alliance. 2024. PMID: 38955468 Free PMC article.
-
LGP2 synergy with MDA5 in RLR-mediated RNA recognition and antiviral signaling.Cytokine. 2015 Aug;74(2):198-206. doi: 10.1016/j.cyto.2015.02.010. Epub 2015 Mar 18. Cytokine. 2015. PMID: 25794939 Free PMC article. Review.
References
Publication types
MeSH terms
Substances
Grants and funding
LinkOut - more resources
Full Text Sources
Other Literature Sources
Molecular Biology Databases